the Creative Commons Attribution 4.0 License.
the Creative Commons Attribution 4.0 License.
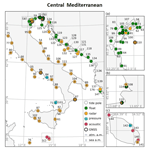
Coastal sea level monitoring in the Mediterranean and Black seas
Begoña Pérez Gómez
Ivica Vilibić
Jadranka Šepić
Iva Međugorac
Matjaž Ličer
Laurent Testut
Claire Fraboul
Marta Marcos
Hassen Abdellaoui
Enrique Álvarez Fanjul
Darko Barbalić
Benjamín Casas
Antonio Castaño-Tierno
Srđan Čupić
Aldo Drago
María Angeles Fraile
Daniele A. Galliano
Adam Gauci
Branislav Gloginja
Víctor Martín Guijarro
Maja Jeromel
Marcos Larrad Revuelto
Ayah Lazar
Ibrahim Haktan Keskin
Igor Medvedev
Abdelkader Menassri
Mohamed Aïssa Meslem
Hrvoje Mihanović
Sara Morucci
Dragos Niculescu
José Manuel Quijano de Benito
Josep Pascual
Atanas Palazov
Marco Picone
Fabio Raicich
Mohamed Said
Jordi Salat
Erdinc Sezen
Mehmet Simav
Georgios Sylaios
Elena Tel
Joaquín Tintoré
Klodian Zaimi
George Zodiatis
Employed for over a century, the traditional way of monitoring sea level variability by tide gauges – in combination with modern observational techniques like satellite altimetry – is an inevitable ingredient in sea level studies over the climate scales and in coastal seas. The development of the instrumentation, remote data acquisition, processing, and archiving in the last decades has allowed the extension of the applications to a variety of users and coastal hazard managers. The Mediterranean and Black seas are examples of such a transition – while having a long tradition of sea level observations with several records spanning over a century, the number of modern tide gauge stations is growing rapidly, with data available both in real time and as a research product at different time resolutions. As no comprehensive survey of the tide gauge networks has been carried out recently in these basins, the aim of this paper is to map the existing coastal sea level monitoring infrastructures and the respective data availability. The survey encompasses a description of major monitoring networks in the Mediterranean and Black seas and their characteristics, including the type of sea level sensors, measuring resolutions, data availability, and existence of ancillary measurements, altogether collecting information about 240 presently operational tide gauge stations. The availability of the Mediterranean and Black seas sea level data in the global and European sea level repositories has been also screened and classified following their sampling interval and level of quality check, pointing to the necessity of harmonization of the data available with different metadata and series in different repositories. Finally, an assessment of the networks' capabilities for their use in different sea level applications has been done, with recommendations that might mitigate the bottlenecks and ensure further development of the networks in a coordinated way, a critical need in the era of human-induced climate changes and sea level rise.
- Article
(8424 KB) - Full-text XML
-
Supplement
(305 KB) - BibTeX
- EndNote
- Included in Encyclopedia of Geosciences
Coastal sea levels have been monitored for decades by networks of tide gauges in ports and harbours, established by a diverse range of institutions to fulfil their specific needs and requirements. Tidal predictions, datum definition, and port operations were the original motivation for creating most of these networks. However, tide gauge data are also needed to understand sea level changes at different spatial and temporal scales and are used by experts in fields such as oceanography, hydrography, meteorology, geodesy, or seismology. The sea level sensors, which measure water level height relative to land with a high accuracy and high temporal resolution (1–60 min), are essential for monitoring and studying coastal sea level hazards that may threaten the coastal strip during episodes of extreme sea levels and coastal flooding, the latter being a combination of storm surges, tsunamis, meteotsunamis, and infragravity waves occurring on top of ongoing sea level rise (Pugh and Woodworth, 2014). Improved knowledge and assessment of sea level changes in magnitude and frequency is essential for coastal planning as well as for establishing early warning systems, for which tide gauges are a key element along with other in situ measurements and forecasting models.
All mentioned hazards are present in the Mediterranean and the Black Sea (M/BS hereafter) and pose a threat to densely populated coastal areas, cultural heritage and historical cities lying near the shore (Fig. 1) (Reimann et al., 2018). This is particularly relevant for some regions exposed to substantial sea level variations spanning a range of frequencies (Fig. 1), from minutes (like meteotsunamis or tsunamis) to hours, days, weeks (storm surges or planetary wave forcing), and seasonal oscillations to interannual variability and decadal trends (Pugh and Woodworth, 2014).
As the Mediterranean and Black seas are microtidal basins (Tsimplis et al., 1995), the atmospherically driven component of sea level (storm surge) is often the most common cause of extreme coastal sea levels. Conjoined with wind-generated waves and/or intense precipitation (also related to increased river discharge), flood risks during a storm surge may lead to devastating flooding events (Bevacqua et al., 2019). According to Cid et al. (2016), the Tunisian (Gulf of Gabes), Aegean, and Adriatic coasts undergo the highest number of sea level extreme events per year. In the Black Sea, the western coast is the most exposed to storm surges (Bresson et al., 2018). Low-elevation areas, deltas and sinking land areas (e.g. the Venice Lagoon and Po Delta in Italy, the Nile Delta in Egypt, the Ebro Delta in Spain) are also subjected to the high flood risks during extreme events (Ferrarin et al., 2021; El-Fishawi, 1989; Hereher, 2015; Grases et al., 2020). Consequently, coastal zone management and protection bodies carry out extensive sea level measurements to support coastal flooding forecasts and issue timely alerts to the population, like the Tide Monitoring and Forecast Centre of the City of Venice that maintains a network comprising tens of tide gauges. From time to time, exceptionally high sea levels in the Adriatic Sea threaten this city in particular (acqua alta phenomenon), causing severe flooding and disruption of people's lives. As an example, on 12 November 2019, the sea level reached 1.89 m (∼ 1.3 m surge contribution), the second-highest storm surge since the 1966 event (1.94 m) (Ferrarin et al., 2021). Occasionally, in other areas, less extreme storm surge events (∼0.5 m), in combination with waves, are also able to cause substantial damage to infrastructure, coastal erosion, and flooding episodes (e.g. the storm Gloria hitting the Spanish Mediterranean coast in January 2020: Amores et al., 2020; Pérez Gómez et al., 2021).
Tsunamis are amplified coastal sea level oscillations with periods ranging from minutes to hours, mainly generated by strong submarine earthquakes. The convergence of the African and Eurasian plates makes them a likely hazard in the Mediterranean (Tinti and Maramai, 1996; Tinti et al., 2001; Papadopoulos and Papageorgiou, 2012; Papadopoulos et al., 2014; Maramai et al., 2014, 2019; Samaras et al., 2015), where around 10 % of all tsunamis worldwide occur, being particularly destructive in the Hellenic Arc area (Fig. 1b). The earthquakes can reach a magnitude of 7.5 to 8 there, triggering >5–6 m wave heights. The hazard is lower in the western Mediterranean Sea (MS), but tsunami waves of over 1 m can reach most of the M/BS locations (Sørensen et al., 2012; Álvarez-Gómez et al., 2011). A well-known ancient tsunami is the one generated by a strong earthquake (magnitude 8–8.5) off Crete in 365 CE, which caused many deaths and much damage in the Middle East and all the way up the Adriatic Sea including the destruction of the Alexandrian port and library (event t4 in Fig. 1b). Also, in Greece, around 1600 BCE a giant tsunami triggered by the collapse of the Santorini volcano is considered to have caused the end of the Minoan civilization in Crete (event t5 in Fig. 1b). Tsunamis can also be generated by landslides and volcanic eruptions, but these events are mostly localized (e.g. Stromboli volcano, Tinti et al., 2005). Recent tsunami events have been recorded by tide gauges: the 9 July 1956 Amorgos 12 m high tsunami (Okal et al., 2009); the 21 May 2003 Algerian tsunami reaching the Balearic Islands' shores as a 2 m wave (Alasset et al., 2006; Vela et al., 2014); and the 20 July 2017 Bodrum Peninsula and the 30 October 2020 Samos Island tsunamis (Dogan et al., 2017, 2019). These records became a valuable source of information for improving tsunami models and therefore for establishing and improving tsunami early warning services. As the tsunami consequences for the coastal population might be disastrous in terms of loss of life and economic damage, several regional tsunami service providers and national tsunami warning systems have been established in the area in recent years (e.g. Schindelé et al., 2015). These systems, capable of assessing tsunami travel time and providing early warnings to civil-protection authorities for vulnerable coastal populations, make use of real-time tide gauge networks, for which a 1 min or less sampling interval is required.
Meteorological tsunamis (also referred to as meteotsunamis) are atmospherically generated long ocean waves that have spectral properties similar to those of tsunami waves and which occasionally – at certain locations – reach the destructive heights of tsunami waves (Monserrat et al., 2006; Rabinovich, 2020; Gusiakov, 2021). The Mediterranean Sea is considered to be a meteotsunami hot spot, i.e. a basin where a destructive meteotsunami occurs once in 1 decade or more often (Vilibić et al., 2021). The most researched Mediterranean meteotsunamis occur in Ciutadella harbour on Menorca Island (Jansà and Ramis, 2021), several locations along the eastern coast of the Adriatic Sea (Orlić, 2015; Orlić and Šepić, 2019), and at Mazara del Vallo on Sicily (Zemunik et al., 2021a). The Mediterranean meteotsunamis are commonly generated by high-frequency (T<1 h) atmospheric gravity waves that, through the Proudman resonance (Proudman, 1929), generate long ocean waves while travelling over shelves. The strongest Mediterranean events typically occur during the summer months (Vilibić et al., 2021) when synoptic situations that favour the generation and propagation of atmospheric gravity waves are more common. Several attempts to construct a reliable meteotsunami warning system for the Mediterranean have been made, all for the Balearic Islands and the Adriatic Sea: these warning systems are designed to have at least one of the following: (i) real-time monitoring of the atmosphere–ocean conditions (Marcos et al., 2009a; Šepić and Vilibić, 2011); (ii) numerical modelling of atmospheric and ocean processes (Denamiel et al., 2019a; Romero et al., 2019), (iii) assessment of forecasted synoptic conditions (Jansà et al., 2007; Šepić et al., 2016b), or (iv) a combination of some of the above (Denamiel et al., 2019b).
Sea level rise, a key indicator of ongoing climate changes is an underlying threat to some coastal areas in the M/BS, particularly to those already exposed to extreme events and low-elevation areas (especially if accompanied by subsiding land). Despite the small number of stations with sufficiently long time series for climate studies, historical monthly mean sea levels from tide gauges have been used to compute coastal sea level trends (Zerbini et al., 1996; Tsimplis and Spencer, 1997; Gomis et al., 2012). These data provide relative sea level trends, relevant for assessing flooding risk and improving coastal protection, which often differ significantly from one place to another due to local land movements (El-Geziry and Said, 2020). Absolute sea level trends can be obtained for those tide gauge stations that are collocated with permanent Global Navigation Satellite System (GNSS) stations that provide the vertical land motion (VLM) correction. Tsimplis et al. (2005) found tide gauge sea level trends of just −0.4 to 0.7 mm yr−1 between 1958 and 2001 in the Mediterranean, revealing slower sea level rise than is the global average for the period. This appears to be due to a negative trend of the atmospheric (storm surge) component, which in turn was caused by a prolonged positive phase of the North Atlantic Oscillation (NAO). However, the Mediterranean sea level trends have increased significantly since the 1990s. For the Mediterranean Basin, Bonaduce et al. (2016) found a mean sea level positive trend of 2.44 ± 0.5 mm yr−1, based on satellite altimetry and tide gauge data for the period 1993–2012. Taibi and Haddad (2019) used 18 tide gauges in the region with data spanning the period of 1993–2015 and found significant trends ranging from 1.48 to 8.72 mm yr−1, after VLM correction, pointing to large spatial differences in the sea level rise.
The majority of the quoted research relies strongly on the tide gauge data, either directly or through their use in the calibration of satellite altimeters. Near-real-time data transmission, combined with a progressive upgrade to a shorter temporal sampling step, have allowed the integration of tide gauge data in storm surge, and tsunami warning systems over the last 15 years. Tide gauge data are also required for the validation of global, regional, and coastal circulation and tsunami models, coastal engineering, or altimetry data calibration. Their multi-purpose and multidisciplinary character is an advantage for the sustainability of the system, ensuring permanent funding in some cases. However, it also presents challenges for basin, regional, and global-scale network coordination initiatives and for data exchange between existing international programmes. This is particularly the case in the M/BS, where restrictive national data policies of some countries, especially along the Mediterranean coast of Africa, have yielded a spatial distribution of stations with available data biased towards the northern countries (Tsimplis and Spencer, 1997; Woodworth et al., 2009). Several attempts in the past have tried to solve this situation: in 1997, the International Commission for the Scientific Exploration of the Mediterranean Sea (CIESM) and the Intergovernmental Oceanographic Commission from UNESCO (IOC/UNESCO) agreed to cooperate in the research on sea level changes in the M/BS by establishing a long-term monitoring network system named MedGLOSS, connected to the Global Sea Level Observing System (GLOSS) (Rosen and Aarup, 2002, https://www.ciesm.org/marine/programs/medgloss.htm, last access: 18 June 2022). The programme, very active between 2001 and 2005, fostered the establishment of a network in the region, supporting data contribution to international programmes, digitization of old chart records, support with quality control, and even the installation and calibration of stations in some countries. Despite the fact that the number of stations has increased significantly in recent years and that the networks in many countries have been modernized (with modernization mainly driven by new requirements of tsunami warning systems), there is still an important lack of available data along the southern coast of the basin. Other more recent initiatives launched in the framework of the Mediterranean Oceanography Network for the Global Ocean Observing System (MONGOOS) or the IOC/UNESCO have also failed, up to now, to fill this gap. Some national sea level networks were substantially upgraded recently following technological development (e.g. the Spanish networks: Pérez Gómez et al., 2013, 2014; Italian, French, and Croatian radar networks), providing the data to users following FAIR (findable, accessible, interoperable, and reusable) principles recently established as the standard in science (Wilkinson et al., 2016). Since 2014, the Joint Research Centre (JRC) of the European Commission has developed and installed a low-cost solution for tsunami and storm surge applications, the Inexpensive Device for Sea Level (IDSL), jointly operated now with several institutions in the region.
A cohesive mapping of in situ coastal sea level monitoring capacities in the M/BS basins has not been available for a long time, (and it has only been done sporadically at national levels, e.g. Vilibić et al., 2005), exceeding the timescale of the technological developments that are rapidly changing the observational landscape in geosciences in general (Le Traon, 2013). The aim of this paper is to assess coastal sea level monitoring capacities at national and basin-scale levels, to survey the availability of sea level data, to address the appropriateness of the networks for the most relevant sea level applications, and to identify required upgrades, maintenance problems, and potential fields of regional cooperation. To achieve this, a survey of coastal sea level infrastructure was conducted in 2021, including most of the relevant institutions operating tide gauges in the region. Results of the survey are presented, including information on the type of technology, ancillary measurements, collocation with GNSS, data sampling and latency, long-term data availability, quality control, and funding status. The initiative enabled us to access relevant metadata and reach national contacts and to improve communication and the exchange of experiences between national experts in sea level studies and tide gauge operators. The survey has been complemented with an assessment on data availability in different international data portals and programmes targeting different applications and an analysis of the fit-for-purpose status of the network based on data availability in those areas more threatened by storm surges, tsunamis, meteotsunamis, and sea level rise. Following the introductory section, Sect. 2 comprises a description of the tide gauge networks or stations operated by the different contributors and presents the main results of the survey. Section 3 is dedicated to data availability in existing international programmes, databases, and data repositories. Section 4 provides an assessment of the network to fulfil targeted applications, while Sect. 5 summarizes the work with several conclusions and recommendations.
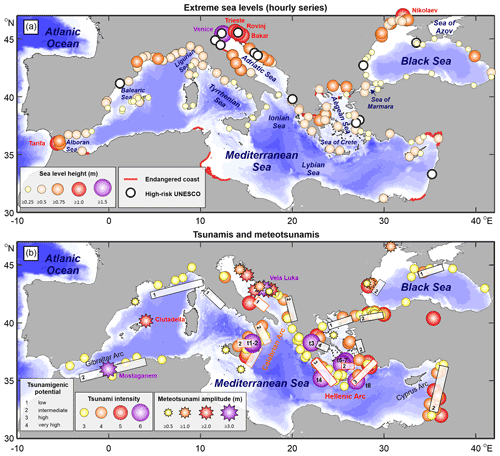
Figure 1(a) Maximum measured sea level heights (with respect to a local mean), based on the GESLA Version 3 dataset and available national databases, are given (coloured circles); strips of coast (red lines) and UNESCO heritage sites (white circles) endangered by present and future-day sea level rise and erosion (after Reimann et al., 2018). (b) Epicentres of earthquakes which resulted in historic tsunamis having intensities of 3 or higher (coloured circles, after Maramai et al., 2014); tsunamigenic fault areas are depicted by white rectangles, and tsunamigenic potential is indicated by the numbers 1–4 (after Fokaefs and Papadopoulos, 2007, and Oaie et al., 2016); locations of historic meteotsunamis surpassing an amplitude of 0.5 m are marked with coloured stars (after Vilibić et al., 2010, 2016; Orlić, 2015; Šepić et al., 2018; Okal, 2021). For extreme hourly sea levels and meteotsunamis, locations at which the most extreme events occurred are named; for tsunamis, the epicentres of earthquakes leading to the eight most destructive tsunamis are marked with t1–t8.
Operating institutions and operators of tide gauge networks and observing sites in the M/BS region have been identified and contacted throughout 2021. We have received input from 30 different agencies, resulting in brief network descriptions, a list of station details (Appendix), and additional questionnaire responses (Supplement).
2.1 Network description
2.1.1 Ports of Spain REDMAR network
The REDMAR network, composed today of 41 multi-purpose radar stations, was established by the Spanish harbour authorities and Ports of Spain (Puertos del Estado: PdE) in 1992, as an aid to port operations and coastal and harbour engineers. The first three stations on the Mediterranean coast were installed that year at Barcelona, Valencia, and Málaga, based on acoustic sensors. Two new stations based on pressure sensors were deployed at Ibiza and Motril in 2003 and 2004, respectively. Ibiza was the first one collocated with a GNSS permanent station, in the framework of the European Sea Level Service Research Infrastructure (ESEAS-RI) project, for altimetry calibration (Martínez-Benjamín et al., 2004).
Today, 17 stations are operated along the Spanish Mediterranean coast, including 1 at Melilla (northern Africa) and 5 in the Balearic Islands. Between 2005 and 2009 all the old stations were upgraded to radar sensors (and 1 min sampling and latency), after an overlapping period of around 1 year to connect the old and the new time series and refer them to the same datum (Pérez Gómez et al., 2014). Ancillary atmospheric pressure and wind data (1 min resolution) are measured at 12 of the 17 stations, and 7 of the stations are today collocated with a permanent GNSS receiver: Barcelona, Tarragona, Ibiza, Mallorca, Almería, Melilla and Tarifa. Data are displayed through the PdE visualization tool (Portus: https://portus.puertos.es/?locale=en#/, last access: 18 June 2022).
All the stations transmit 1 min data with 1 min latency to PdE, the National Geographic Institute (National Tsunami Warning System) and the IOC Sea Level Station Monitoring Facility (IOC-SLSMF). Automatic quality control and processing is applied every 15 min for integration in the multi-model sea level forecasting system (Pérez Gómez et al., 2021). In addition, 2 Hz raw data are processed every hour to characterize higher-frequency sea level oscillations with periods of minutes (García-Valdecasas et al., 2021). Delayed-mode quality control and processing are performed annually, and monthly mean sea levels are sent to the Permanent Service for Mean Sea Level (PSMSL). The data are also available through the Copernicus Marine Service In Situ Thematic Assembly Centre (Copernicus Marine Service In Situ TAC), the EMODnet (European Marine Observation and Data Network) portal, and the Global Extreme Sea Level Analysis (GESLA) dataset. Mediterranean stations operated by PdE are listed in Table A1 in the Appendix.
2.1.2 SOCIB tide gauge network in the Balearic Islands, Spain
The SOCIB (Balearic Islands Coastal Observing and Forecasting System) tide gauge network (Tintoré et al., 2013, 2019) was established in 2009 and currently consists of six stations around the Balearic Islands, five of which are located on Mallorca and one on Ibiza. The length of the nearly continuous tide gauge records varies between 5 and 12 years, with the shortest series dating back to 2016. With the exception of the tide gauge in Sant Antoni (Ibiza), which is a radar gauge, the rest are pressure gauges. The sampling frequency is 1 min for all tide gauges. Together with sea level, all stations measure atmospheric pressure, sampled every 30 s, and five of them also monitor water temperature every minute. All data are freely distributed in near-real time through the SOCIB website and are also available through the Copernicus Marine Service In Situ TAC data portal. Sea level observations are referenced to the tide gauge benchmarks, whose positions are controlled on a yearly basis through GNSS surveys. Stations operated by SOCIB are listed in Table A2.
2.1.3 Spanish National Geographic Institute tide gauge network
The tide gauge network of the National Geographic Institute of Spain (IGN) has a set of sensors that gather changes and variations in the mean sea level over time. It started in the 19th century, when three tide gauges were set up in order to determine the national altimetry datum in Alicante, Santander, and Cádiz. The purpose was to establish the required infrastructure to start levelling works. The tide gauge network has been extended and its instruments have been improved since then, including recent upgrades from float to radar sensors. Nowadays IGN has 10 tide gauges: 5 are located in the Iberian Peninsula, 1 on Alboran Island, and 4 in the Canary Islands. Only five are on the Spanish Mediterranean coast. All of them have one or two radar sensors and are linked to GNSS permanent stations. A sea level dataset starting in 1870 has been recently published for Alicante, based on data from historic float gauges and modern radar sensors at this harbour (Marcos et al., 2021). The Mediterranean part of the network is complemented by five IDSL sensors provided by the JRC in Cartagena, La Mola de Mahón, Ciutadella and Ceuta.
In addition to maintenance work, network management, and connection to High Precision Levelling Network (REDNAP), IGN analyses the historical series of its tide gauges. It also compares mean sea level changes with GNSS observations, among which a new technique stands out. It is called Global Navigation Satellite System interferometric reflectometry (GNSS-IR), and it is still under study. Mediterranean stations operated by IGN are listed in Table A3.
2.1.4 Spanish Institute of Oceanography tide gauge network
The sea level data network operated by the Spanish Institute of Oceanography (Instituto Español de Oceanografía: IEO) consists of 11 stations: 6 on the Iberian Peninsula, 1 at Ceuta on the northern Africa coast, 1 in the Balearic Islands, and 3 in the Canary Islands. For historical operative reasons, most of these locations are those in which IEO local headquarters are located. Each tide gauge station is equipped with two sensors: an analogue float-type tide gauge with a digital encoder and a radar-based one.
The analogue network is one of the oldest ones in Spain, with some of the measurements dating back to 1943. Historical data are made available through the SeaDataNet data portal (https://www.seadatanet.org/, last access: 18 June 2022). Each of the analogue sensors consists of a float gauge, mechanically connected to an analogue–digital encoder, which converts the data for an on-site computer. The radar sensors duplicate the measurements and ensure measurement continuity when one of the two devices fails. The 1 min sampled data are stored locally and recovered via modem by the central data centre once a day, where the data quality is assessed and archived.
The routines for data recovery, quality assessment, the detection of high-frequency events, and data representation are currently being updated, and it is expected that the frequency of data availability will reach one per minute by the end of the year 2021. Mediterranean stations operated by IEO are listed in Table A4.
2.1.5 Spanish Hydrographic Office
Currently, the Spanish Hydrographic Office (IHM) is making a great effort in the installation of a permanent tide gauge network along the national coast. Usually, the IHM instals tide gauges in the hydrographic work area on a temporary basis to be able to calculate the real or reduced probe data. Once the work is finished, the tide gauge is usually removed.
Since 2021, the IHM has installed permanent tide gauges in different ports on the coast: Rosas, Castellón (on the Mediterranean coast), and Huelva (in the Gulf of Cádiz). The intention is to install eight new tide gauges during 2022, with the objective to further increase the density of tide gauges along the Spanish coast. The equipment consists of acoustic sensors with a frequency of 1 Hz and with spatial positioning in real time through a GNSS receiver. At the moment, the web is under development. Mediterranean stations operated by IHM are listed in Table A5.
2.1.6 L'Estartit tide gauge (Meteolestartit, in collaboration with ICM/CSIC-Spain)
The tide gauge is a part of the Meteorological and Oceanographic Station (Meteolestartit) in the harbour of L'Estartit, a coastal town on the Catalan coast, in the NW Mediterranean. It is a float type or analogue tide gauge which records sea level measurements on paper. Recordings are collected every week and digitized with a 2 h resolution. Paper records are preserved for further detailed analyses if required in some special circumstances, such as seiches. The position of the tide gauge is georeferenced every 5 years by the Catalan Cartographic Institute, and sea level data are linearly corrected backwards for each period. Sea level record collection at this point started in January 1990, as part of Meteolestartit, which started in 1969, as a personal initiative of Josep Pascual with the collaboration of the Marine Sciences Research Institute in Barcelona (ICM/CSIC). Data collected included basic meteorological and oceanographic data. More details can be found in Pascual and Salat (2019) and Salat et al. (2019). Details about L'Estartit tide gauge are in Table A6.
2.1.7 SHOM tide gauge network RONIM, France
French Naval Hydrographic and Oceanographic Service (SHOM) has been observing the tides for many years, but the RONIM network, as it exists today, was initiated in 1992, mainly to meet the needs of tide prediction and reduction of bathymetric surveys. It was based on a network which was already in place and which consisted, in 1996, of five tide gauges. It was then densified to reach 23 tide gauges in 2007, including 5 in the Mediterranean (Marseille, Toulon, Nice, Monaco, and Ajaccio). Some tide gauges were installed at sites where SHOM already had sea level observations, allowing for the continuation of measurements and a prolongation of time series. At that time, the Mediterranean tide gauges were equipped with acoustic sensors.
Today, the RONIM network consists of 50 stations of which 14 are currently active in the Mediterranean Sea. All tide gauges are equipped with radar sensors and also have an atmospheric pressure sensor. In the Mediterranean, three tide gauges are collocated with a GNSS (Sète, Marseille, and Toulon). Most of the tide gauges are equipped with a double real-time transmission system: internet and satellite. In the Mediterranean, all tide gauges except for Marseille are equipped with satellite transmission. The acquisition rate is 1 Hz, and these data are sent directly to SHOM and the tsunami warning service by a virtual private network (VPN) link.
The 1 min data are computed at SHOM and transmitted in near-real time to the data.shom.fr website and to the IOC-SLSMF website with a latency of 5 min. The satellite messages are clocked every 6 min. The data transmitted by satellite are also on the IOC-SLSMF website. As there is a double transmission system, on the IOC-SLSMF site, the stations are given twice (e.g. Toulon (internet transmission) and Toulon2 (satellite transmission)). The 10 min data are calculated by the tide gauge data logger. They are retrieved once a day and transmitted to the data.shom.fr website. In addition, the RONIM network data are also available on the Copernicus Marine Service In Situ TAC, EMODnet, GESLA dataset, SONEL (GLOSS GNSS data assembly centre), and PSMSL data portals.
A major upgrade of the RONIM network is underway (2021/2022). The data logger and the real-time transmission process will be replaced, and two additional sensors (webcam + meteo station) will be added at most of the tide gauges. A unique supervision system will be implemented for all tide gauges, allowing for a better assessment of real-time recording and transmission issues and an improvement of the network reliability. Mediterranean stations operated by SHOM are listed in Table A7.
2.1.8 ISPRA tide gauge networks along the Italian coast
The Italian Institute for Environmental Protection and Research (ISPRA) comprehensively and systematically provides high-resolution estimates for the physical state of the Italian seas as well as real-time monitoring at national and local levels. The marine observation system includes two sea level measurement networks: the Italian Tide Gauge Network (Rete Mareografica Nazionale – RMN), which continuously monitors the sea level and a number of related meteorological and physical parameters, and the North Adriatic and Venice Lagoon Tide Gauge Network (Rete Mareografica della Laguna di Venezia e del Litorale Nord Adriatico – RMLV), which is used for the real-time storm surge prediction and warning system. Some IDSL stations were also tested, but the only one still operational is in Marina di Teulada.
The RMN network is a crucial source of information related to sea level. It provides data useful for analysing sea level variations, predicting storm surges and developing a tsunami early warning system. The RMN consists of 36 measuring stations uniformly distributed along the Italian coast, mainly located within harbours. Some of these stations have been operating since the 1970s. The tidal-wave measurements for the entire network are provided by two different instruments (radar and float) and can be simply configured by a remote command. Each measurement station is also equipped with meteorological sensors: an anemometer (wind speed and direction at 10 m above ground level), a barometric sensor, and a multiparametric sensor for humidity and temperature, which are necessary for the real-time evaluation of sea and weather conditions. Four stations (Venezia, Crotone, Gaeta, Carloforte) are collocated with GNSS stations, to detect the horizontal and vertical displacement of the cabin.
The RMLV network is composed of 26 tide gauge stations equipped for the systematic and widespread measurement of water level and other related parameters, such as wind direction, wind speed, atmospheric pressure, precipitation, and wave heights in the Lagoon of Venice and along the north Adriatic coastline. Lots of the RMLV stations have been operating for several decades and Venezia – Punta della Salute station for more than 150 years. Real-time data represent one of the main utilities, fundamental for prediction and warnings of exceptional or atypical high tides (storm surges). Two RMLV stations (Venezia Punta della Salute and Grado) are collocated with GNSS in order to detect the continuous vertical shift of the local zero tide level, which is the reference benchmark for tide measurements in the Lagoon of Venice (ZMPS). The real-time operability of this network is crucial for several purposes, such as the analysis and elaboration of data referring in particular to extreme events (storm surges), signalling, and forecasting exceptional high tides. Moreover, data from the Venice Lagoon and north Adriatic tide gauge network (RMLV) are an important source for planning Venice's defence from the phenomenon of high tides and for scientific studies on sea level variations.
Quality-control procedures have been implemented in order to validate sea level historical series and to guarantee data compliance with international standards. Furthermore, automatic quality control procedures are applied to real-time data. Data are distributed through ISPRA portals (http://dati.isprambiente.it (last access: 18 June 2022), https://www.mareografico.it/ (last access: 18 June 2022), https://www.venezia.isprambiente.it/ (last access: 18 June 2022) and also https://tsunami.isprambiente.it/ (last access: 18 June 2022), which is powered by the JRC Tsunami Alert Device server software described in the next section) and through international initiatives (IOC-SLSMF, EMODnet, MONGOOS, EuroGOOS). Stations operated by ISPRA are listed in Table A8.
2.1.9 The Joint Research Centre (JRC) IDSL network
As already mentioned in Sect. 1, acknowledging the low quantity of sensors deployed on the Mediterranean coasts, the JRC started investigating the adoption of retail technology in 2014, in order to produce a low-cost solution. Since 2014, with the adoption of a few custom components, the reliability of the Inexpensive Device for Sea Level (IDSL) measurements further evolved retaining its best characteristics: low-cost (about EUR 2000), high-frequency (5 s), and local intelligence (detection of anomalous sea oscillations through assessing deviation from moving averages).
Funded by the IOC/UNESCO, three campaigns delivered devices throughout the Mediterranean, the Black Sea, and the north-east Atlantic. Another collaboration led to providing Indonesia with six IDSL devices. All data collected by the IDSL network are available online through the Tsunami Alert Device (TAD) server on the JRC website. Stations operated in Italy by the JRC with ISPRA are listed in Table A9. The JRC also operates these sensors in collaboration with other institutions in other countries, such as Greece (Table A17), Türkiye (Table A22), Cyprus (Table A23), Lebanon, and Morocco (Table A27).
2.1.10 The tide gauge station of Trieste, Molo Sartorio, Italy
The Italian National Research Council (CNR) operates one station through the Trieste branch of the Institute of Marine Sciences (CNR-ISMAR). The station, included in the GLOSS Core Network with no. 340, is located at Molo (Pier) Sartorio, in the harbour of Trieste, and it is equipped with two float instruments with a digital encoder, and one 50-year old fully analogue tide gauge. Direct sea level measurements are performed at least twice a month to check the instrument stability. The data quality control is performed in delayed mode at least once a year.
The tide gauge cabin also hosts two barometers, one of which is digital and one analogue. A GNSS receiver, operated by the University of Bologna, is mounted on top of the building that includes the tide gauge cabin.
The earliest sea level measurements were made in 1859, and since then the tide gauge has remained on the same pier; more details can be found in Zerbini et al. (2017). Unfortunately, from 1875 to 1939, only monthly mean sea levels are available (with a few gaps); they can be retrieved from the Permanent Service for Mean Sea Level (PSMSL) data bank. Hourly data are available for 1939 onwards (Raicich, 2019), while 1 min data exist since 2001. Hourly means since June 2009 are available as Fast Delivery data from the University of Hawaii Sea Level Center (UHSLC). The review of pre-1939 data including the digitization of the available charts is in progress. Details about the station Trieste Molo Sartorio are in Table A10.
2.1.11 The mareographic station in Koper, Slovenia
Operational sea level monitoring in Slovenia began in 1958 with the construction of the tide gauge station in Koper, for which hourly sea level measurements since 1961 are available. The station is operated by the Slovenian Environment Agency and is collocated with the GNSS station. The existing float-type sensor in a stilling well was upgraded in 2005 with an additional radar sea level sensor, having 1 mm accuracy and 10 min sampling time. It provides sea level data, sea temperature data at 1 m of depth, GNSS data, and essential meteorological data (air pressure, wind, air temperature, relative humidity, solar irradiance). The quality control is automatic, while additional manual controlling of sea level measurements is performed weekly at the station location. Details about the station Koper are in Table A11.
2.1.12 Croatian tide gauge network
The Croatian tide gauge network is operated by the Andrija Mohorovičić Geophysical Institute (AMGI), the Hydrographic Institute of the Republic of Croatia (HHI), the Institute of Oceanography and Fisheries (IOF), the National Agency for Water Management Hrvatske vode (Croatian Waters, HV), and the Ruđer Bošković Institute (RBI). The network consists of permanent stations based on float-type technology installed in stilling wells, established during the 20th century, and of radar-type stations installed from 2004 onward.
Systematic measurements of sea level in Croatia, based on float-type stations, started in 1929 when the AMGI (Zagreb) established a tide gauge station in Bakar. In the same year, sea level measurements were initiated in Split Harbour by the HHI, but the station was destroyed by bombing in World War II. During the 1950s four long-term coastal stations were installed: Dubrovnik (1955), Split Harbour (1955) and Rovinj (1955) by the HHI, and Split Marjan (1956) by the IOF. The network was again extended when the HHI installed stations Zadar in 1990 and Ploče in 2002, and HV installed stations near the Mala Neretva river mouth in 1977, at Prosika in 1986, and at Golubinka in 1995. Most of the network was modernized in the early 2000s by mounting analogue–digital converters to floats, along with GSM (Global System for Mobile Communications) modems for real-time/near-real-time data acquisition. Modernization was mostly done in the framework of the ESEAS-RI project (EU Framework Programme 5). In 2004 an open-air radar sensor was installed at Bakar station and GNSS at Split Harbour station. Ancillary atmospheric pressure (Bakar and Split Marjan) and wind (Split Marjan) measurements are carried out too. Most of the stations store 1 min data locally and transmit them with up to 15 min latency to home institutions. Exceptions are the stations Golubinka and Prosika, from which data are collected on site at least once a year. At most stations digital data are automatically quality checked, processed, and displayed (http://geo101.gfz.hr/~bakar/index_files/ (last access: 18 June 2022), https://adriaticsea.hhi.hr/site/login (last access: 18 June 2022), http://vodostaji.voda.hr/, last access: 18 June 2022) in real-time mode or once a day (Bakar), while analogue data are digitized at an hourly resolution and mostly processed once a year. The maintenance of stations includes regular checks of recorder zero (mainly twice a year), cleaning of the stilling well and connecting pipe (once in 2 to 5 years), and levelling of the contact-point level against tide gauge benchmarks. All stations are levelled towards the national geodetic datum. Most time series have shorter gaps due to various reasons. Monthly and yearly averages from the AMGI, HHI, and IOF stations have been sent to the PSMSL since the stations were established.
Since the 1950s, additional portable float-type chart-recording tide gauges were occasionally operational along the Croatian coast of the Adriatic, with corresponding sea level measurements spanning periods from 1 to 18 years, depending on location: Vis and Ušće Neretve (measurements during 1957), Mali Ston (1957–1959), Broce (1957–1959), Ubli (1987–1991), Sućuraj (1987–2005), Žirje (1989–1991), Zlarin (1983–1988), Gaženica (1983–1988), and Rijeka (1998–1999).
The Croatian tide gauge network was expanded in 2017–2021 with nine new coastal stations based on radar technology. Stations in Stari Grad (Hvar Island), Sobra (Mljet Island), and Vela Luka (Korčula Island) were installed in 2017 (within the framework of the MESSI (Meteotsunamis, destructive long ocean waves in the tsunami frequency band: from observations and simulations towards a warning system) and POZOR (Monitoring of potentially dangerous sea level oscillations and their contribution to floods of coastal areas in the future climate) projects) and are operated by the IOF. Station Šibenik, located in Sv. Ante Channel was established in 2020 and is operated by the RBI. In 2021 the IOF established the stations Vis (Vis Island), Mali Lošinj (Lošinj Island), Bistrina (Mali Ston Bay), and Raslina (Lake Prokljan – ocean station) as part of the Interreg Italy – Croatia projects ECOSS, CHANGE WE CARE, and RESPONSe. Also, in 2021 the HHI deployed a radar sensor in Rijeka near the traffic port. All instruments are installed in the open air providing 1 min data, which are averages of 1 s measurements done for 20 s during each minute. All stations locally store these 1 min data and transmit them with up to 10 min latency to home institutions. The exception is Šibenik, at which data are stored with a temporal resolution of 55 min. Ancillary atmospheric pressure and wind are measured at Stari Grad, Vela Luka, Mali Lošinj, Vis, and Bistrina (1 min data), while surface current and sea temperature (depths of 0.5, 1.0, 2.0, and 4.0 m) are measured at Šibenik station (every 55 min). Tide gauges in Rijeka, Stari Grad, Vela Luka, and Sobra are levelled to the national geodetic datum. By the end of 2022, stations Rijeka and Sobra are planned to be upgraded with ancillary meteorological sensors. The Vela Luka tide gauge was temporarily decommissioned in June 2021, but it should be reinstalled during 2022. All non-quality-controlled sea level and atmospheric data from the IOF stations are available directly through the IOF website (http://faust.izor.hr/autodatapub/mjesustdohvatpod?jezik=eng, last access: 18 June 2022). In addition, sea level data measured at Sobra, Stari Grad, and Vela Luka are also available through the IOC-SLSMF website (since October 2018). Data from Rijeka and Šibenik are visualized at https://adriaticsea.hhi.hr/site/login (last access: 18 June 2022) and https://hv.geolux-radars.com/sites/sibenik-svante.html (last access: 18 June 2022), respectively.
In addition, the HHI plans to install two additional radar-type tide gauges in the following years in the areas of Šibenik and Mali Lošinj. Stations operated by Croatian institutions are listed in Table A12.
2.1.13 Montenegrin tide gauge network
The Institute of Hydrometeorology and Seismology of Montenegro (Department of Hydrography, Podgorica, Montenegro) is responsible for the monitoring and maintenance of the tide gauges installed in the Montenegrin part of the Adriatic Sea. The network includes two permanent tide gauge stations based on float-type technology. The tide station in Bar was established in 1965 and till 1991 was part of the former Yugoslavia tide gauge network (Slovenia, Croatia, Montenegro). During the 1990s the tide station in Bar was not fully operational for a long time, but it has been restored and re-connected to the national geodetic network through levelling. The second Montenegrin tide gauge station was established in Kotor in 2010. For both stations the sea level is measured once every 6 min, with GSM-based data retrieval to the central server. Stations operated by Montenegro are listed in Table A13.
2.1.14 Tide monitoring network in Albania
The Institute of GeoSciences, Department of Hydrology, of the Polytechnic University of Tirana is responsible for monitoring all water parameters in Albania, including the sea level. The sea level observations are taken manually, two times a day: at 07:00 and 19:00 UTC. These data are stored in a book by the observer and sent every month to the Institute of GeoSciences (Tirana, Albania). At the centre, this information is archived and not controlled, unless there is a request for these data. Stations operated by Albania are listed in Table A14.
2.1.15 Sea level observations in the Maltese Archipelago
The routine collection of sea level data in the Maltese Archipelago was initiated in May 1993 by the Physical Oceanography Unit (later Physical Oceanography Research Group) using an ENDECO-type 1029/1150 differential pressure gauge in Mellieha Bay on the northern coast of Malta. The station remained in operation until 2001, measuring in delayed mode every 2 min, supplemented with meteorological measurements collected at a nearby station in Ramla tal-Bir overlooking the southern Comino Channel. This endeavour was mainly intended to assess the sea level variability and to study the phenomenology of strong seiches locally known as the “milghuba” (Drago, 2000, 2009).
A MedGLOSS station installed in February 2001 in the Portomaso marina at the Malta Hilton in St. Julians constituted the first real-time ocean observing system in Malta. The instrument, donated by the International Commission for the Scientific Exploration of the Mediterranean Sea (CIESM), collected sea level data every 30 s and also seawater temperature, atmospheric pressure, and waves in the marina. Hourly averaged observations were shared in real time with the MedGLOSS network through the Israel Oceanographic and Limnological Research (IOLR) that coordinated the project. The system comprised an underwater Paroscientific pressure sensor, a Digiquartz Intelligent sensor, and a Setra atmospheric pressure sensor. In 2010, the system was upgraded with new equipment to enable higher sampling rates and to enable a first phase towards contributing to the Mediterranean Tsunami Warning System within NEAMTWS (North-Eastern Atlantic, the Mediterranean and Connected Seas Tsunami Warning and Mitigation System).
More recently, in collaboration with the JRC, the sea level observing network has been enhanced by two IDSL stations, set up at Delimara (March 2021) and Senglea (June 2021). Data are transmitted in real time to the University of Malta as well as to the JRC-TAD server (https://webcritech.jrc.ec.europa.eu/TAD_server/Device/555, last access: 18 June 2022) with a temporal frequency of 5 s. Each station is equipped with a radar sensor connected to electronics that also measures air temperature and captures visual images of the sea state every 15 min.
In March 2021, a Radac WaveGuide sensor was installed at the tip of the Marsaxlokk breakwater to measure sea level, wave height, and wave period. The instrument was procured through the SIMIT-THARSY project, partially funded by the European Commission European Regional Development Fund through the Operational Programme. This radar sea level gauge is capable of measuring water displacement with a resolution of 3 mm at a frequency of 10 Hz. The wave height is measured with an accuracy of 1 cm at 1 min intervals. In front of it, on the other end of the bay, another IDSL was deployed. The operational data from these stations are linked to other observations and delivered in real time through the PORTO stations web interface developed through the CALYPSO South project, another Interreg V-A Italy–Malta project (https://www.calypsosouth.eu/, last access: 18 June 2022). Tide gauges operated by the Physical Oceanography Research Group of the University of Malta are listed in Table A15.
2.1.16 Greek tide gauge network operated by the Hellenic Navy Hydrographic Service
The Hellenic Navy Hydrographic Service (HNHS) monitors sea level variability over the Aegean and the Ionian seas through a network of 22 tide gauges. All hydrographic stations consist of analogue float-type gauges equipped with rotating drums, rotating with a speed of 1 cm h−1. These tidal stations are located within port facilities to record sea level continuously and provide these measurements to the relevant port authorities. The tidal stations in Thessaloniki, Kavala, and Piraeus are the oldest of the network, operating continuously since 1933.
Since 1990, HNHS upgraded the network by installing digital water level sensors at seven selected stations (Piraeus, Alexandroupolis, Kalamata, Katakolo, Lefkada, Siros and Chios), operating in parallel to the analogue systems. These stations collect, store, and transfer water level data to central servers in near-real-time mode. Digital stations additionally collect a series of ancillary parameters, like the sea surface temperature and meteorological data (air temperature, humidity, barometric pressure, and wind speed and direction). In parallel, during that period, the network's tidal data, recorded in paper charts, have been digitized as hourly values and subsequently organized and stored in the HNHS databases. The water level error is estimated to be approximately 1 cm (Tsimplis, 1994).
Data from four stations (Piraeus, Katakolo, Siros and Kalamata) are visualized online through the HNHS web page (https://www.hnhs.gr/en/online-2/tide-graphs, last access: 18 June 202). Data from all tidal gauges covering the 1969–2020 period are also transferred to the Permanent Service for Mean Sea level (PSMSL), supported by the IOC/UNESCO (Bitharis et al., 2017). Sea level data from these four digital stations are also provided and visualized in real-time mode to the IOC-SLSMF. Stations operated by HNHS are listed in Table A16.
2.1.17 Bulgarian coastal sea level service
Systematic sea level measurements were initiated in Bulgaria in the beginning of the 20th century, with 16 tide gauge stations operational over a certain period of time till 2013. Operators of these sea level stations were as follows: the National Institute of Meteorology and Hydrology, Bulgarian Academy of Sciences (NIMH) – six stations; Cadastre Agency, Ministry of Regional Development and Public Works (CA) – four stations; Port Infrastructure (PI) – five stations; and Institute of Oceanology, Bulgarian Academy of Sciences (IO-BAS) – one station. At present, five of these stations are operated jointly by the Institute of Oceanology, National Institute of Geophysics, Geodesy and Geography, and the Geodesy, Cartography and Cadastre Agency and provide data in real time. The stations are equipped with a high-accuracy radar instrument. Active Bulgarian stations are listed in Table A18.
2.1.18 National Institute for Marine Research and Development (NIMRD) “Grigore Antipa” coastal sea level monitoring, Romania
Sea level measurements started in Romania in 1856, on the initiative of the European Danube Commission. However, these data have not been preserved. Regular recordings of the sea level started in Romania in 1933, by installing a float gauge. Presently, two methods of measurements are used in Constanţa: a pressure sensor and a float gauge, with a hydrometric sight, at which visual measurements are performed three times a day for data quality purposes. The accuracy of the analogue measurements on a paper chart are estimated to 1 mm. At Sulina and Mangalia, the measurements are made using pressure sensors. The sea level data are transmitted to the server via the GPRS/GSM (General Packet Radio Service/Global System for Mobile Communications) method.
In Sulina, Mangalia, and Constanţa three IDSLs were deployed in the last decade. Presently, only the one in Mangalia is operational. Stations operated by NIMRD “Grigore Antipa” are listed in Table A19.
2.1.19 Black Sea stations operated by the All-Russian Research Institute of Hydrometeorological Information – World Data Center
Sea level observations on the north–north-eastern coast of the Black Sea began with the first Russian stations in the middle of the 19th century. Systematic measurements of the sea level were initiated in 1873. Since 1944, the sea level network was restored, reconstructed, and expanded. Sea level float-type recorders were installed at many tide gauges. Since 1977, all tide gauges have been tuned to a single system of heights (the Baltic height system). In total, over the years, in the territory of the Soviet Union on the Black Sea, there were 44 tide gauges, the data from which were saved until 1985. For 23 tide gauges, there are long-term digital series of hourly observations of sea level. Today short-period sea level variations are measured at five tide gauges: Tuapse, Sochi, Sevastopol, Yalta, and Feodosia. The Tuapse tide gauge is collocated with a GNSS. The Russian tide gauge network is owned by the Russian Federal Service for Hydrometeorology and Environmental Monitoring (Roshydromet) and the mentioned stations are operated by the All-Russian Research Institute of Hydrometeorological Information – World Data Center (listed in Table A20).
2.1.20 Coastal sea level monitoring in Türkiye
Sea level monitoring activities in Türkiye date back to the mid-1930s, when the first float-type tide gauge was installed at Antalya harbour to determine the national vertical datum. Since then, a considerable number of temporary mechanical and analogue tide gauges has been deployed in different spots. In 1999 the Turkish National Sea Level Monitoring System (TUDES) programme was initiated by the General Directorate of Mapping. Up to 2011, under this programme, a network consisting of 20 digital tide gauges with acoustic sounding tubes, for continuous measurements, was established. Due to the significant maintenance problems related to the acoustic gauges and the strong need for VLM monitoring, all TUDES stations were replaced with radar gauges after 2015, and most are GNSS collocated. The data averaged at 30 s and 15 min intervals are transmitted to the data centre in Ankara in near-real time through GSM and the internet. Real-time and delayed-mode quality controls, data analysis, database management, and data distribution activities are performed at the data centre. Further, TUDES delivers sea level data to the regional networks (e.g. NEAMTWS). More information about the TUDES can be found at https://tudes.harita.gov.tr/?lang=us (last access: 18 June 2022).
In addition, three IDSL stations were deployed in Bozcaada, Samsun and Bodrum. TUDES tide gauge stations are listed in Table A21, while Table A22 contains the JRC IDSL stations in Türkiye operated by the Kandilli Observatory and Earthquake Research Institute (KOERI).
2.1.21 Cyprus tide gauge networks
The systematic monitoring and transmitting of real-time sea level data in Cyprus was first initiated in 2001 as part of the MedGLOSS Mediterranean sea level network. Initially, one tide gauge was deployed at Paphos harbour in 2001 by the Department of Fisheries and Marine Research Oceanography Unit (DFMR) and hourly data were transmitted along with atmospheric pressure and in situ sea temperature via the internet to a dedicated MedGLOSS web page hosted by the Marine Data Center of the IOLR. Later, this station became part of the MedGLOSS/ESEAS and continued to transmit data until 2015. After the December 2004 catastrophic Indian Ocean tsunami, the Paphos sea level station was also set to transmit sea level data in real time to the IOC-SLSMF every 1 min. Moreover, the station was included in the coastal sea level monitoring network of the NEAMTWS (North-Eastern Atlantic, the Mediterranean and Connected Seas Tsunami Warning and Mitigation System; UNESCO, 2007).
In the framework of MedGLOSS/ESEAS activities, another three tide gauges were deployed in Cyprus during 2010, at the Zygi and Paralimni fishing shelters and in the Larnaca marina. The tide gauge deployed at the Zygi fishing shelter was provided by the Cyprus Governmental Department of Lands and Surveys. These tide gauges were operated only for 2 years, between 2010–2012, the data of which, together with those from the Paphos station (operated between 2001–2015), were used to estimate the lowest astronomical tide and the lowest low tide at the locations of their deployment (Papazachariou, 2014). Furthermore, studies were carried out to examine the trend of the sea level variations at the Paphos station (Loizides et al., 2010) as well as inter-comparison of the Paphos time series data with satellite altimetry time series (Banks et al., 2003; Papazachariou et al., 2014)
The Paphos MedGLOSS/ESEAS tide gauge was equipped with a Paroscientific Digiquartz Intelligent pressure sensor pressure type, while the remaining three Cyprus MedGLOSS/ESEAS tide gauges were equipped with AANDERAA pressure sensors.
In the framework of the Interreg THALCHOR2 project, a new tide gauge network named PYTHEAS has been deployed in Cyprus during 2018 (Danezis et al., 2020). The PYTHEAS tide gauge network consists of four stations (Paralimni and Pomos fishing shelters and Paphos and Larnaca harbours), all owned by the Cyprus Governmental Department of Lands and Surveys (DLS), while the fifth one (old Limassol harbour) is owned by the Cyprus University of Technology. All the PYTHEAS sea level networks were equipped with radar tide gauge sensors, along with atmospheric sensors, such as pressure, air temperature, humidity, and sea temperature. The station at the old Limassol harbour was also set to serve as a GNSS reference. The data from the PYTHEAS tide gauge network are transmitted in real time to the hydrographic database of the DLS.
In parallel and independent from the PYTHEAS network, an additional radar tide gauge station was deployed at the end of March 2018 by the European JRC (Joint Research Centre) at the Zygi fishing shelter, next to the location of the Cyprus MedGLOSS/ESEAS Zygi station. The 5 s data from this station are provided in real time also to the IOC-SLSMF (http://www.ioc-sealevelmonitoring.org/station.php?code=zygi1, last access: 18 June 2022).
In 2019, an offshore station for sea level variation was deployed in the framework of the Interreg HERMES project using a bottom-mounted AWAC ADCP (acoustic Doppler current profiler) pressure sensor. The ADCP was deployed at a water depth of 40 m in Larnaca Bay, close to the famous scuba diver shipwreck Zenobia. In addition to sea level variation, the ADCP measures sea currents at 20 water depths, waves, sea temperature, and suspended particles. The data are transmitted in real time via cable from the bottom-mounted ADCP to a connected surface oceanographic buoy and then via a GPRS to the ORION (Joint Research and Development Centre, Cyprus) server for use on the dedicated HERMES web page (Zhuk et al., 2020). Cyprus stations are listed in Table A23.
2.1.22 Israel tide gauge network
The National Institute of Oceanography, Israel Oceanographic and Limnological Research (IOLR) operates two tide gauges based on pressure sensors located at Hadera (since 1992) and Ashkelon (since 2012). Both stations include other sensors and provide ancillary measurements of several meteorological and oceanographic parameters. The station of Hadera is GLOSS station no. 80. In addition, the JRC deployed three IDSL sensors for tsunami warning at Ashdod, Hadera, and Haifa in February 2018, but only the latter is still operational today. More details of the tide gauges in Israel are provided in Table A24.
2.1.23 Egyptian tide gauge network
Six tide gauges were operational along the Egyptian Mediterranean coast. These gauges were deployed at Port Said, Burullus's new harbour, Abu-Qir Bay, Alexandria Western Harbour, Sidi Abdel-Rahman, and Mersa Matrouh. The periods of data availability are different for each location, with the longest records (30 years) at Alexandria Western Harbour and the shortest records (4 years) at Mersa Matrouh.
In June 2018, in collaboration with the JRC, the National Institute of Oceanography and Fisheries (NIOF) deployed an IDSL station in the port of Alexandria. Stations of the Egyptian tide gauge network are listed in Table A25.
2.1.24 Algerian tide gauge network
The National Institute of Cartography and Remote Sensing (INCT) is responsible for equipping the national territory with all kinds of geodetic networks: GNSS, gravity, and levelling networks. Historically, the altitudes of the Algerian levelling network are reported to several origins generally chosen in an arbitrary manner, with the level deduced from the indications of the tide gauge of La Goulette (Tunisia), the altitude of the landmark of Porte De France (Tunisia), or the coast of La Goulette (Tunisia), or the console placed at Sidi El Hemessi station (Tunisia) in 1914.
Being aware of the significance in providing the national territory with a precise altimetric reference, the INCT put effort into the installation of automatic tide gauges along the Algerian coasts, first to bring the bathymetric surveys to a stable reference, hydrographic zero, or nautical chart zero and then to predict the tide or define reference levels. In addition, the INCT is in charge of setting up the hydrographic zero as an altimetric reference for water heights and uses any tidal data as a national reference (official journal): general knowledge of the tide, determination of harmonic constants, and extreme levels and tidal prediction.
The installation of six tide gauge stations with automatic acquisitions along the Algerian coasts (Ghazaouet, Oran, Ténès, Algiers, Jijel, and Annaba) in the mid-2010s upgraded the network substantially, in particular for monitoring sea level variations and for the modernization of the national altimetric reference. Currently, the INCT is additionally upgrading the network through setting up permanent GNSS stations in collocation with the tide gauge stations at the ports of Algiers, Jijel, Oran, Annaba, Ghazaouet and Ténès. This approach would constitute an important phase for the creation of a multi-observation platform for spatial measurements, gravity field, levelling, and tide gauge. An upgrade of observatories to have real-time data acquisition is also planned for the near future. Stations of the Algerian tide gauge network are listed in Table A26.
2.2 Summary of the existing coastal sea level monitoring infrastructure
With the aim of facilitating a more general assessment of the coastal sea level monitoring networks individually described in Sect. 2.1, a brief questionnaire survey was conducted, resulting with information on (i) institution, country, and contact name of a network; (ii) the number of stations; (iii) the main purpose of the network; (iv) the funding mechanism; (v) data policy; (vi) the raw time sampling interval; (vii) data latency; (viii) the number of sea level sensors at each station; (ix) the type of tide gauge; (x) the levelling strategy; (xi) the number of tide gauges collocated with a GNSS; xiii) ancillary measurements; (xiv) quality control and processing; and (xv) public data availability. The survey responses are tabulated and may be found in the Supplement.
Based on the responses received, 240 active stations have been identified so far in the M/BS. These are operated by 30 agencies, representing most countries in the region except for Morocco, Tunisia (both have sea level networks, e.g. Jabnoun and Harzallah, 2020), Libya, Bosnia and Herzegovina, Ukraine, Georgia, Lebanon, and Syria. We could also not get information from the Italian Hydrographic Office, which operates the Genoa tide gauge, one of the longest-operating Mediterranean tide gauges. In some countries tide gauges are operated by several institutions (e.g. six in Spain, five in Croatia), while others have one national network managed by a single agency (e.g. SHOM, in France). ISPRA in Italy runs the largest network in terms of the number of stations (62), followed by the JRC (24 tsunami stations operated jointly with other institutions), HNHS in Greece (23), and the TUDES Turkish network (20). The majority of respondents are responsible for a smaller number of stations, even for just one single station in several cases.
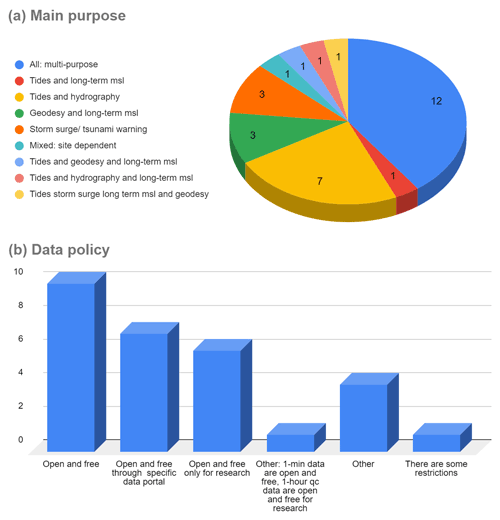
Figure 2(a) Primary objective of the coastal sea level monitoring networks based on responses to the survey and (b) data policy of the operating institutions as collected by the survey.
Figure 2a displays the primary objective of the networks, chosen by operating institutions between the following non-exclusive choices: (i) tides and hydrography; (ii) storm surge/tsunami warning; (iii) geodesy and long-term mean sea level (MSL); (iv) tides and long-term MSL; (v) altimetry calibration; (vi) model validation; (vii) harbour operation and aid to navigation; (viii) meteotsunamis; or (xi) multi-purpose (all previous options). Most of the networks, especially the largest ones, are claimed to be multi-purpose (40 % of answers: 12) followed by those mainly aimed at tides and hydrography applications (23 % of the answers: 7), such as the HNHS Greek network, Romania, and one of the Cyprus networks. Three institutions reported that their stations are focused on storm surge/tsunami warning and another three on geodesy and long-term MSL. None of the stations have been specifically implemented for applications (v)–(viii). The IOLR network in Israel provided a mixed, site-dependent answer, depending on technology and time sampling and latency (e.g. the two radar sensors installed for storm surge/tsunami warning). Despite more than half of the answers claiming open and free access to data (altogether 10 agencies and 7 agencies through specific data portals), there are also some data policy issues (Fig. 2b): open and free only for research were selected by six respondents (+1 for 1 h quality-controlled data), while five institutions mentioned unspecified issues or restrictions on data access.
With respect to network sustainability related to maintenance strategy and status, most of the respondents confirm no problems of funding now or in the near future. Problems with maintenance are reported by NIOF in Egypt and for a couple of stations in Croatia (IOF) and Israel (IOLR). The most serious issues are raised by the Albanian network, which is not being maintained at this moment, and there are no plans for funding in the short term. The JRC also warns that the network funding is guaranteed now, but it might stop at any time. All the agencies rely on their own resources for in situ maintenance except for PdE (Spain), ISPRA (Italy), and Croatian Waters (Croatia), which subcontract this work.
The raw time sampling interval is 1 min for most of the networks in the region (12 of the respondents). However, a large range of raw sampling options are provided by the rest of contributors, from 6.7 Hz resampled to 5 s (JRC tsunami stations) to 55 min (Ruđer Bošković Institute), 1 h (Croatian Waters), or 2 h (Meteolestartit). Some agencies are more specific in the answer to this question and report several samplings available depending on data portal or application. The latency of data transmission is claimed to be real time ( min) by nine of the respondents (IOLR only for the two radar sensors), near-real time (minutes, hours or days) by 15 of them, and the delayed-mode access only by 7 agencies.
One of the most challenging aspects of a tide gauge network is datum stability and link to other official references, especially for long-term and mean sea level studies. According to the responses, the majority of networks perform high-precision connection of the tide gauge benchmark (TGBM) to the national geodetic datum as the basic levelling strategy. In some cases, they provide the date of the last levelling campaigns, but in general there is a lack of information about their frequency, apart from periodic levelling near the TGBM during routine maintenance reported by four institutions (usually once per year). Connection of all stations to a nearby GNSS station is reported by the Spanish IGN and by the Algerian network.
With respect to quality control and data processing, the response is also diverse, as shown in Fig. 3. In most cases only delayed-mode quality control is performed by data originators (17 respondents, 6 of which also generate sea level products). In addition to delayed-mode quality control and products, automatic quality control in near-real time is also performed nowadays by seven agencies in the area, while only two agencies do not perform quality control or processing at all.
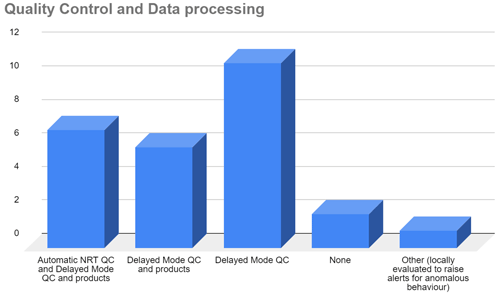
Figure 3Quality control and data processing strategies followed by M/BS tide gauge network operating agencies.
The list of stations including name, coordinates, data period and ancillary measurements is provided in the Appendix (Tables A1–A27). This list is the basis for detail mapping of existing sea level infrastructure in the M/BS, composed of 240 active stations, including the type of sensor (radar, acoustic, float, or pressure sensor), ancillary meteorological or oceanographic data, or collocation with a nearby permanent GNSS station (Figs. 4–6). The data periods on this table reveal that the oldest active stations in the M/BS are as follows: Marseille, 1849–present (France); Trieste Molo Sartorio, 1875–1889; 1901–present (Italy); Burgas port, 1910–present (Bulgaria); Varna port, 1919–present (Bulgaria); Venice Punta della Salute, 1924–present (Italy); Alicante 1, 1928–present (Spain); and Bakar, 1929–present (Croatia). These stations, the most relevant for climate research, started with float gauges and have been upgraded to radar sensors in most cases. Very often, time series from different tide gauges at the same harbour are used to generate a dataset spanning the whole history of the station (e.g. the new Alicante dataset by Marcos et al., 2021, starting with first measurements by a tide pole in 1870).
In terms of technology, Figs. 4–6 reveal that today the radar sensors are the most common type of instrument in the network, a sea level sensor being present at 134 stations (56 %) and dominating especially in the Western Mediterranean. Further, 69 stations (29 %) are still based on, or combined with, traditional float gauges, mainly along the eastern Adriatic coast and in Greece. Acoustic sensors are used at 27 locations (mostly tsunami sensors), while pressure sensors are used at 15 stations (Balearic Islands, Western Black Sea, and Israel). At four stations sea level measurements are still performed manually using a tide pole (Albania).
Only nine stations have two different sensors: float and radar (Algeciras, Ceuta, Tarifa, Koper, and Bakar), float and pressure (Constanţa), and float and acoustic (Algiers, Jijel, and Oran). The GLOSS recommendation for tsunamigenic areas is to use a radar plus a pressure sensor, a combination not found in the M/BS. Promising news is the increasing number of stations collocated with a GNSS and therefore with potential geocentric reference (TGBM ellipsoidal height) and VLM information: there are 55 locations (23 % of the network), with 27 in the Western Mediterranean, 8 in the central Mediterranean, 10 in the Eastern Mediterranean, 3 in the Marmara Sea, and 7 in the Black Sea. At 154 stations (64 % of active stations) there are ancillary sensors which provide meteorological data (84 stations, mainly atmospheric pressure and wind), oceanographic data (15 stations), or both (55 stations, many of them in the central Mediterranean).
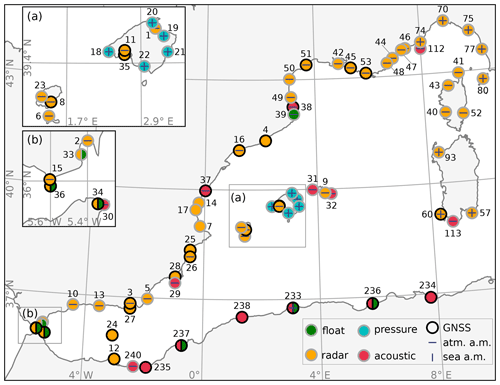
Figure 4Active tide gauge stations in the western Mediterranean Sea. Marker colour indicates tide gauge sensor, while black circle indicates collocation with GNSS station. Ancillary measurements, atmospheric or oceanographic, are indicated by navy blue horizontal and vertical dashes, respectively. Station names are listed in the Appendix tables: Tables A1–A9 and A26–A27. Stations 26, 27, 29, 32, 34, 35, 36, and 112 are slightly shifted to ensure better visibility.
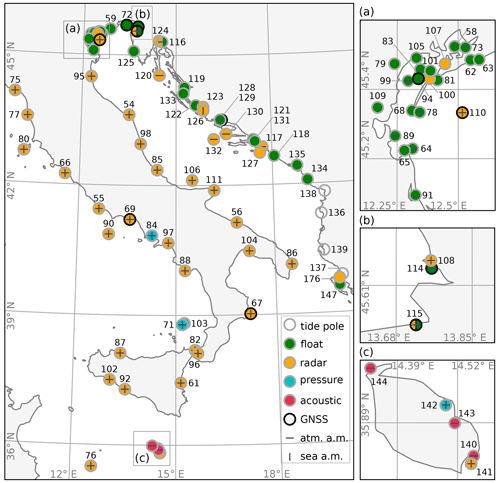
Figure 5Same as in Fig. 4 but for the central Mediterranean Sea. Station names are listed in the Appendix tables: Tables A8 and A10–A17. Stations 82, 103, 114, 121, 123, 124, 126, and 128 are slightly shifted to ensure better visibility. Some stations appear to be inland due to the relatively coarse resolution of the coastline.
In this chapter, we assess the M/BS sea level data availability. The assessment is primarily based on the public global and European data repositories as of 15 November 2021, which have been established for various applications (from real-time applications to research-quality products) and contain data of different time sampling steps (from 1 min to 1 month). Figures 7 and 8 summarize information on the presented data repositories.
3.1 Monthly sea level data repository
The oldest sea level data repository, at which monthly sea level data have been collected for almost a century, is the Permanent Service for Mean Sea Level (PSMSL, https://www.psmsl.org/, last access: 18 June 2022). The PSMSL was established in 1933 by Joseph Proudman, with the aim of collecting, analysing, and interpreting monthly sea level data at the global scale (Woodworth and Player, 1993; Holgate et al., 2013). The collected data are of high quality and freely available, thus providing an excellent dataset for mean sea level studies in this era of climate changes (e.g. Spada and Galassi, 2012). In total, there are 158 M/BS data series with a high reliability (classified as Revised Local Reference (RLR) data) (Fig. 8a), with 6 records spanning more than 100 years. These stations are Trieste, Marseille, and Genoa in the Mediterranean, with the respective length of 146, 136, and 114 years and data coverage of 87 %, 97 %, and 78 %; then come Poti, Batumi, and Tuapse stations in the Black Sea (145, 137, and 103 years of length, respectively, and data coverage of 94 %, 87 %, and 99 %, respectively). Due to their exceptional length, these series have been thoroughly checked (e.g. Tsimplis and Spencer, 1997; Wöppelmann et al., 2014) and used in numerous long-term sea level assessment studies (e.g. Letetrel et al., 2010; Pashova, 2012).
In the PSMSL repository, there are 38 tide gauge stations with monthly records longer than 50 years, concentrated mostly along the northern coastlines of the M/BS (Fig. 8a). Along the north African coast there are long-term (50+) data from only two stations, Alexandria and Ceuta, making proper quantification of sea level changes along the southern Mediterranean challenging (e.g. Gomis et al., 2012). At a number of sites (24 of them) there are two or more tide gauges collocated, with the long-term data coming from the float-type tide gauges and with additional sea level series normally coming from the digital instruments (radar, acoustic or pressure tide gauges) and spanning the last few decades at best. The median data coverage of the M/BS PSMSL series is 92 %, while 63 stations have a data coverage higher than 95 %. There are 19 sea level records which have 100 % data coverage, but all of them are relatively short records (up to 30 years) – these series mostly come from digital instruments. Oppositely, there are four sea level records with data coverage of less than 50 %, with three of them containing data from the 1960s and 1970s.
3.2 Hourly sea level data repositories
At hourly resolutions, some sea level records coming from the PSMSL tide gauges are also available in the Copernicus Marine Service In Situ TAC (http://www.marineinsitu.eu, last access: 18 June 2022), a service providing operational data-driven ocean products plus systematic information on the ocean and sea-ice state (Le Traon et al., 2019). The Copernicus Marine Service In Situ TAC database contains hourly sea level records from 115 tide gauges in the M/BS (Fig. 8b), with the median length of the series being 2 years. Hourly sea level data records can also be found in the EMODnet Physics in situ observations repository (Novellino et al., 2015, https://www.emodnet-physics.eu/map, last access: 18 June 2022), which is developed to be a single point of access to near-real-time and historical ocean data in Europe. The repository contains hourly sea level observations from 119 tide gauges in the two basins (Fig. 8b). For some stations, the data are available both from recent observations and from historical periods, sometimes of the same length or even longer than the PSMSL records but mostly covering shorter periods and sometimes without any data available for download – these “empty” stations are not included here. There are 12 hourly sea level series in the EMODnet repository longer than 30 years, while more than half of the stations have records 4 years long or shorter.
In conjunction with EMODnet Physics and the Copernicus Marine Service In Situ TAC, the relevant research product for the M/BS, containing sea level data at an hourly resolution, is the Global Extreme Sea Level Analysis dataset (GESLA, http://www.gesla.org (last access: 18 June 2022), Woodworth et al., 2016, 2017) – here version 3 is analysed. GESLA is a research-driven sea level product containing series of hourly or higher sampling resolution, which encompass 1355 sea level records and 39151 station years all over the world. In the M/BS, GESLA lists 101 stations, most of them spanning periods much shorter than listed in the PSMSL (up to a few decades). The longest GESLA record in the Mediterranean is the one for Marseille (France) tide gauge, 173 years long, while the median length of GESLA records is 15 years.
In addition to the quoted centralized data repositories, some of hourly sea level data can also be accessed through the SeaDataNet portal (https://www.seadatanet.org, last access: 18 June 2022), which is a virtual centre that provides different ocean metadata, data and products archived by data providers. However, the access to the sea level archives is not straight-forward. It is mostly distributed towards data providers; sea level data are often combined with data from other observing platforms (e.g. echo sounders, synthetic aperture radars) and data are also provided in different formats and split into small observing intervals, making this portal rather complicated to use. Still, some data not listed in the EMODnet Physics, Copernicus Marine Service, and GESLA dataset may be found there, like sea level records for the Georgian, Ukrainian, and Maltese stations.
It is a challenge to a researcher to choose the best database out of the three and to locate research-quality data. In various databases, data originating from the same location frequently have different names, identification numbers, and metadata. We thus encourage the founders of these data repositories to join their data and to provide one high-quality dataset for further research, with unique data policies allowing for accessibility of the data and following the FAIR (findable, accessible, interoperable, and reusable) principles (Wilkinson et al., 2016).
3.3 Minute sea level data repositories
Another important sea level data portal is the UNESCO Intergovernmental Oceanographic Commission Sea Level Station Monitoring Facility (IOC-SLSMF, http://www.ioc-sealevelmonitoring.org, last access: 18 June 2022), which – contrary to other sea level data repositories – has not been developed for collection of research-quality data but for operational purposes (Aarup et al., 2019). The IOC-SLSMF has been developed to deliver the information about the status of tide gauges operating in real time as well as to visualize the data downstreaming to the service (Flanders Marine Institute (VLIZ), 2021). Further, the service provides high-resolution (mostly with a 1 min resolution) sea level data at a global level, following the demands of the operational and early warning systems that emerged after the 2004 Indian Ocean tsunami.
As of 15 of November 2021, the IOC-SLSMF provides information and data from 143 tide gauge stations in the M/BS, most of which (all but 30 for the preceding week) are operational in real time with a data transmitting latency between 1 and 10 min (Fig. 8c). Ten stations have been operational since 2008, when they were installed as a result of activities of the IOC Intergovernmental Coordination Group for the North-Eastern Atlantic, the Mediterranean and Connected Seas Tsunami Warning and Mitigation System (IOC ICG/NEAMTWS; Amato, 2020), established to coordinate regionally the development of the tsunami early warning system. The most recent additions to the network are from 2019 and 2020 and are related to a few Spanish, Greek, and Turkish tide gauges. The availability of the IOC-SLSMF data in the Mediterranean is largely dependent on the data providers, which normally upgrade large segments of networks at once. For example, most of the Italian sea level records have been available from December 2013, which is also the median value for the beginning of data provision of all the Mediterranean IOC-SLSMF stations (Zemunik et al., 2021b). Most of the stations from which data are available at the IOC-SLSMF database are equipped with the radar tide gauge technology; sampling rates are normally 1 min, except for Greek and Turkish sea level records that are largely available with the resolutions of 30 and 20 s.
Recently, the European Commission through the JRC at Ispra established a sea level database (https://webcritech.jrc.ec.europa.eu/, last access: 18 June 2022, here marked as JRC-SLD, Fig. 7), containing multi-year records of a 1 to 10 min resolution. The database assembles 105 stations in the M/BS operating in real time, providing also the estimates of tidal constants for each site. In addition, the companion site at https://webcritech.jrc.ec.europa.eu/tad_server/ (last access: 18 June 2022) collects the sea level data at a rate of 5 s, aimed to improve the tsunami hazard monitoring, in particular in the Mediterranean Sea and in the North Atlantic area (NEAMTWS area of IOC/UNESCO).
As no global research-quality sea level data repository containing the data at a 1 min resolution has been developed yet, Zemunik et al. (2021b) applied quality-check procedures to the IOC-SLSMF data and provided the Minute Sea level Analysis product (MISELA), to be used for researching high-frequency sea level phenomena. The MISELA dataset contains 331 sea level records and 2303 station years between 2004 and 2019, with a resolution of 1 min and containing only the high-frequency part of the signal (cut-off period at 2 h). In the M/BS, MISELA quotes 36 stations, covering a time period from 2008 at the earliest up to 2019, with the longest record coming from Melilla and Barcelona, Spain.
3.4 Data providers' sea level data repositories
Aside from the listed global sea level data repositories and research products, these sea level data are also accessible through websites of data providers (e.g. PdE, SOCIB, SHOM, ISPRA; see more in Sect. 2). Further, there are sea level data that are not included in listed repositories, like ISPRA Rete Mareografica Laguna di Venezia, containing sea level records from 24 tide gauge stations, but that are available through local repositories (https://www.venezia.isprambiente.it, last access: 18 June 2022). Last but not least, there are more sea level records, in particular at an hourly timescale, of which the raw data are not easily reachable by users. Some of these tide gauge operators provide access only to recent sea level data and some are visualized through a graphical interface, while others are available on demand – often only for research purposes – and others have even more restrictive data policies.
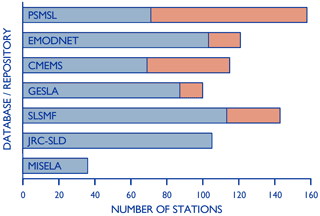
Figure 7Number of tide gauge stations in the Mediterranean and Black Sea for which sea level data can be found in the quoted databases or data repositories. As of 15 November 2021, the stations having no data since 2018 for the PSMSL, since 2021 for EMODnet and the Copernicus Marine Service and since 2020 for GESLA and being not operational in the preceding 7 d for SLSMF and JRC-SLD are indicated in red. For MISELA, data are available up to 2019, at best.
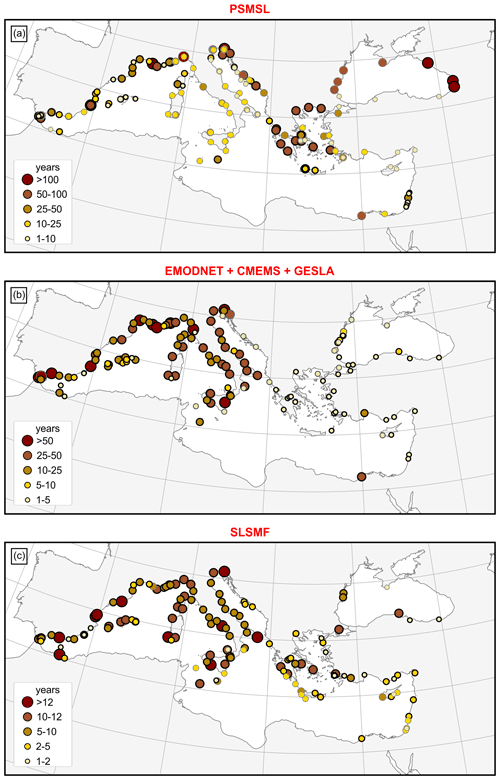
Figure 8The Mediterranean and Black Sea data accessible as (a) monthly sea level averages in the PSMSL (the length of series is indicated by the size and colour of the circle; thick border lines denote the series that end in 2018 or later and grey the series that end earlier), (b) hourly sea level data in EMODnet Physics, the Copernicus Marine Service, or GESLA (the length of series is indicated by the size and colour of the circle; thick and grey lines denote the series that do or do not, contain the data in 2021, respectively), and (c) 1 min series in the IOC-SLSMF (the length of the operability period is indicated by the size and the colour of the circle; thick and grey border lines denote the stations that were or were not operational on 15 November 2021, respectively).
Based on the information compiled in Sects. 2 and 3, an overview of the fit-for-purpose status of the network, to fulfil some specific applications, is presented below. Examples of use of tide gauges in the M/BS are provided, focusing on early warning systems, long-term sea level variability, or altimetry calibration, all in order to show the main gaps or lack of information in terms of coastal in situ measurements.
4.1 Operational, early warning, and forecasting systems
4.1.1 Tide tables and port operations
Historically, the first requests for sea level information came from the needs of navigation near the coasts and access to ports with significant tidal ranges. Tide scales or other visual systems quickly gave way to tide gauges, which became a standard in port operations, in particular at ports with intense traffic and a large tidal range (Lemon, 2003). Although the traffic in the M/BS is substantial, the tides are small and normally range over few tens of centimetres, except in the northern Adriatic and Bay of Gabes (Tsimplis et al., 1995). Nonetheless, several countries produce tide tables to predict the astronomical tide.
The tides are normally predicted using harmonic analysis of measured signal. Sea level observations allow for the estimation of the harmonic constituents necessary to recompose the tidal signal and provide highly precise estimates of the tidal signal. In the tide table, predictions of the times and heights of high and low tides are published for certain points, called reference ports or main ports, for which there are generally long series of observations that allow us to obtain an accurate prediction. Each reference port can be linked to one or more secondary ports. For these secondary ports, predictions are obtained by applying time and distance offsets from the reference port. According to the International Hydrographic Organization (IHO) recommendations, the reference level of the tide/water height observations and predictions for navigators should be the same as the chart datum.
Use of tide gauges for computing tidal harmonic constants and tide predictions, usually done by national hydrographic services, has allowed port authorities throughout the world to programme their operations well in advance. This is particularly important for those ports that receive large cargo ships with a large draft, for which the time of high or low water is critical. In the M/BS, where the tide is often very small, even negligible, tide tables are only used on those areas with higher tidal ranges. This is the case for the northern Adriatic Sea, where port authorities and pilots in Croatia, Slovenia, and Italy use tide tables with this purpose. Tide predictions are not used on some other coastlines with smaller tides: as an example, the Spanish Hydrographic Office does not generate tide tables for Spanish Mediterranean ports. In these cases, shorter-term sea level forecasts based on storm surge models are the main prediction tool available for harbour operators, as is the case for Barcelona harbour, which relies on the forecasting system developed by PdE and described in Sect. 4.1.2. Of course, more precise information can simultaneously be derived from sea level observations transmitted in real time by the tide gauges.
As ships are getting larger and larger, they have an increasingly large draught, leaving little room for manoeuvre for navigation. The precise knowledge of the water level in real time allows the port authorities to optimize the movement of ships. PdE in Spain has developed a harbour visualization tool essential for managing port operations, which integrates real-time tide gauge data with other measurements (e.g. wind) and data from models. Sea level is a key component of a local early warning system for every harbour, which issues alert messages whenever the sea level or high-frequency sea level oscillations above predetermined thresholds occur. These thresholds can be configured by harbour operators. In Croatia, the Hydrographic Institute also developed a web application with real-time and predicted data, so port authorities and pilots can visually see the difference and adjust their operations accordingly. Real-time tide gauge data are also used by the ports during local bathymetric surveys and dredging activities.
The data collected by the sensors all around the world are used by the JRC-SLD, https://webcritech.jrc.ec.europa.eu/ (last access: 18 June 2022) to compute the harmonic coefficients needed to forecast the tide in locations of the IDSL sensors and the surrounding areas. Most of the data collected by the JRC-SLD are analysed with the same software used by the IDSL network to assess the behaviour of the sea level rise and decrease. In the case of an anomaly, this detection disseminates alerting information.
4.1.2 Storm surges and coastal flooding
Apart from high-frequency sea level disturbances (discussed separately in Sect. 4.1.3), the Mediterranean coastal floods typically occur due to constructive superposition of tides and meteorologically induced storm surges. High local precipitation, increased river discharge and waves may further worsen coastal flood risks and lead to compound flooding episodes (Bevacqua et al., 2019). All these events pose a threat to cultural heritage, and densely populated coastal communities of the M/BS.
Storm surges are most severe along the Tunisian, Aegean, and Adriatic coasts, where the highest number of sea level extreme events per year is observed (Cid et al., 2016). Especially critical is the area of the northern Adriatic Sea and Venice Lagoon, exposed to the well-known acqua alta phenomenon. To face these hazards, coastal regions rely on forecasting operational systems (Umgiesser et al., 2021), measurement networks, and extreme-event research activities (Calafat et al., 2014). In the Venice Lagoon, investment in coastal protection through planning and building of flood barriers has been essential.
These challenges are purely operational: short-term day-to-day flood risk needs to be continuously estimated on synoptic timescales using observation-driven numerical forecasting models and other types of early warning systems (Žust et al., 2021; Makris et al., 2021; Ferrarin et al., 2020; Bajo et al., 2019; Mel and Lionello, 2014; Ferrarin et al., 2013; Pérez Gómez et al., 2012, 2021). Ensemble modelling allows dealing with uncertainties by generating probabilistic envelopes of possible sea levels (Bernier and Thompson, 2015; Bertotti et al., 2011; Ferrarin et al., 2020). Of course, different modelling, ensemble, and observation–ingestion paradigms may be used (Calafat et al. 2014). Panoramically speaking, the modelling of sea levels can be classified into numerical physical models on the one hand and deep-learning approaches on the other. Advantages of the former are typically more extensive spatial coverage – which comes at a higher computational cost – and better performance in the extreme tails of sea level distributions. Deep-learning systems on the other hand demonstrate rapid progress in their forecasting capabilities at very low computational cost (once trained) (e.g. Žust et al., 2021).
Operational forecasting sea level models, producing forecasts on the timescales of hours to days, typically cannot resolve low-frequency sea level variability on the timescales of days to weeks (shown to significantly precondition coastal flooding in some parts of the Mediterranean; e.g. Pasarić et al., 2000; Pasarić and Orlić, 2001; Ferrarin et al., 2021) and are further constrained by the limited sea state knowledge at the time of the simulation. Therefore, sea level observations must somehow be introduced into the model during or after runtime. This can be done in several ways. A simple nudging scheme makes use of near-real-time tide gauge data from the last 7 d in the Nivmar storm surge forecasting system run by Ports of Spain since 1998 (Álvarez Fanjul et al., 2001). Another reliable approach to do this is tide gauge data assimilation (e.g. using ensemble Kalman filtering as in Bajo et al., 2019), followed or complemented by statistical bias corrections with reference to real-time sea level data. Deep-learning approaches (e.g. Žust et al., 2021; Bajo and Umgiesser, 2010) on the other hand offer an alternative way of handling sea level observations: providing near-real-time sea level observations together with tidal model forecasts enables deep networks to learn the biases in tidal models and compensate for this in real time without resorting to numerically expensive schemes like data assimilation.
The Adriatic Sea serves as an example where observation-driven ensemble sea level modelling is absolutely imperative for reliable predictions of cyclone-induced wind-driven floods in Venice and other coastal towns along the northern Adriatic coast. Its elongated shape leads to well-defined seiche periods and resonant amplification of tides (Medvedev et al., 2020). Its bathymetry on the other hand leads to topographic amplification of the sea level signal on the northern Adriatic shelf. The total sea level signal in the northern Adriatic therefore critically depends on the mutual reinforcement between storm surge, tides, and seiches, which in turn depend on the temporal phase difference between peak storm surge, peak tide, and peak seiche (see e.g. Cavaleri et al., 2010). High sensitivity to the phase difference between these components is the reason that even minor errors in predicting the storm timing or trajectory may lead to substantial errors in the total sea level forecast (Cavaleri et al., 2020), which makes ensemble modelling a clear advantage.
Focusing on short-term forecasting of the Venice Lagoon sea level, ISPRA developed and manages an integrated system, made up of in situ data coming from the RMN and RMLV networks (see Sect. 2.1.8) and numerical and statistical models. The sea level forecasting system is mainly based on the deterministic SHYFEM (Shallow Water Hydrodynamic Finite Element Model), and it provides sea level forecasts up to 96 h depending on the spatial resolution (40 km in the Mediterranean Sea, 2 km in the Adriatic Sea, 100 m in the Venice Lagoon). It uses ECMWF (European Centre for Medium-Range Weather Forecasts) and BOLAM (Hydrostatic Meteorological Limited Area Model developed by CNR-ISAC (Italian National Research Council, Institute of Atmospheric Sciences and Climate, Bologna)) meteorological fields, as input data, and it assimilates the sea level measured by the 36 RMN tide gauges. This integration and the improvement of both in situ observations and modelling system shows a good example of efficiency and functionality to prevent and mitigate the impact of flooding and meteo-marine extreme events on the Italian coastal environment.
Other operational storm surge forecasting systems have been in place in the Mediterranean Sea for the last 2 decades (Umgiesser et al., 2021). These systems are progressively being improved or combined with existing 3D baroclinic models, new higher-resolution models, and ensemble and multi-model statistical techniques that provide sea level forecasts with a confidence interval (probabilistic forecast). For example, in the western MS, Ports of Spain runs a multi-model storm surge forecast (named ENSURF: Ensemble SURge Forecast: Pérez González et al., 2017; Pérez Gómez et al., 2021) that combines the output of the above-mentioned storm surge forecasting system Nivmar with Copernicus Marine Service circulation models operational in the region today: IBI-MFC (Iberia–Biscay–Ireland Monitoring and Forecasting Centre, Sotillo et al., 2015) and MED-MFC (Mediterranean Monitoring and Forecasting Centre, Clementi et al., 2019). The system employs the Bayesian model average (BMA) statistical technique, for which near-real-time data from the 17 REDMAR tide gauges on this coast (Sect. 2.1.1) are used to generate an improved probabilistic forecast at these harbours. This technique is also a valuable tool for operational validation and detailed assessment of the different operational systems. Another multi-model ensemble forecasting system has also been recently developed for the Adriatic Sea, combining 10 models predicting sea level height (either storm surge or total water level) and 9 predicting waves characteristics (Ferrarin et al., 2020).
On longer timescales, mid- to long-term coastal management and spatial planning indicates a growing need to understand the impacts of climate change and global mean sea level rise on coastal floods in terms of their intensity and frequency on multi-decadal timescales (Međugorac et al., 2021; Oppenheimer et al., 2019; Bonaldo et al., 2019; Lionello et al., 2017; Androulidakis et al., 2015; Šepić et al., 2012; Marcos et al., 2011). Multi-decadal time series of sea level observations are indispensable in available research since they are the only way of pinning model reanalyses (e.g. Escudier et al., 2021) to past sea level variability, thus paving the way for reliable projections.
Several long-term studies (Androulidakis et al., 2015) indicate that different dynamic contributions to the global mean sea level will have to compensate for each other to some extent, but it can nevertheless be claimed with very high confidence that global mean sea level rise will lead to a substantial overall risk increase in coastal extreme events (Oppenheimer et al., 2019). Consequently, a well-functioning sea level observation network will be more and more imperative for synoptic coastal flood forecasting and mitigation in the M/BS.
4.1.3 Tsunamis and meteotsunamis
A carefully planned network of real-time accessible tide gauge stations is a must for efficient research, monitoring and issuing of tsunami and meteotsunami early warnings. The meteotsunami network should, in addition, be supplemented with air pressure and wind sensors.
Tsunamis, like earthquakes, cannot be predicted. Once they are triggered, mostly by submarine earthquakes, they propagate over thousands of kilometres in the ocean and will reach the coastline in a matter of hours or even of minutes for those coastal areas which are closer to the tsunami source. As fast detection is essential, tsunami warning systems must rely on real-time seismological networks (real-time information about the earthquake) and real-time information of sea level height oscillations. The latter are provided by shore-based tide gauges and by offshore buoys with bottom pressure sensors (tsunameters, e.g. Deep-ocean Assessment and Reporting of Tsunamis (DART) buoys). The first alert messages are issued from seismic information. However, assessing the tsunamigenic potential of an earthquake is not easy, so sea level measurements are needed to confirm that a tsunami was generated and to reduce the number of false alarms. Tsunami propagation models are used to forecast the time and amplitude of the wave on arrival at different coastal points and can also be validated with sea level observations. Adequate communications infrastructure allows issuing correct and timely warnings to local emergency management officials, who can decide to activate their emergency protocols to evacuate low-lying coastal areas in advance of the initial tsunami wave.
When the tsunami of 2004 hit the Indian Ocean causing one of the most devastating disasters of our recent history, only the Pacific Ocean had a tsunami warning system in place. Considering how many lives could have been saved, IOC/UNESCO established several intergovernmental working groups for the implementation of regional and national tsunami warning systems in other basins, such as the North-Eastern Atlantic, the Mediterranean and Connected Seas Tsunami Warning and Mitigation System (NEAMTWS; UNESCO/IOC, 2012a). In 2005, most of the tide gauges in the NEAMTWS region were not suitable for tsunami warning. Requirements for this application are less restrictive in terms of accuracy or datum stability than for long-term sea level trends estimates and consist mainly of improving timeliness and lowering sampling intervals to 1 min or less for the adequate measurement of tsunami wave amplitude and arrival time. A basin-wide distribution of stations is needed, with more stations in those areas closer to tsunamigenic sources, as is the case for a significant part of the Mediterranean coast.
Following new NEAMTWS requirements for sea level data exchange, many stations have been upgraded in the M/BS region since 2005 and today provide higher-frequency sea level data in real time to regional and national tsunami warning systems. In addition, the last GLOSS Implementation Plan (UNESCO/IOC, 2012b) suggests that GLOSS Core stations can be configured to support storm surge and tsunami warning systems. This approach has ensured the multi-purpose character of some of the stations, which is good for network sustainability but has raised new challenges on standard quality control and data processing techniques that need to be adapted and the development of automatic tools for tsunami detection (Holgate et al., 2008; Beltrami et al., 2011; Pérez Gómez et al., 2013; UNESCO/IOC, 2020). These stations are handled by national institutions and warning systems, although most of them also contribute to the IOC-SLSMF and the JRC-SLD portals described in Sect. 3 as well.
Based on the data availability on these portals and the response from national institutions to this survey, at least 152 of all active stations in the M/BS are today contributing to tsunami warning systems, including those upgraded and those installed for this purpose (e.g. a total of 24 IDSL stations installed by the JRC and 19 radar stations operated by the National Observatory of Athens (NOA) on the Greek coast). However, only three of these stations are located on the northern African coast: Melilla, Alexandria, and Saidia Marina. The French Tsunami Warning Centre (CENALT) developed tools based on tsunami wave modelling to monitor the existing sea level network capacity for tsunami detection (Schindelé et al., 2008, 2015). As an example, they found that the tsunami generated by an earthquake north of Algeria in 2003 would not be confirmed by the tide gauge network in less than 70 min, when the travel time to the most affected zones was 30–40 min. The tool, which provides guidance for the implementation of additional tide gauges, demonstrated that adding four tide gauges – two in the Balearic Islands, one in Sardinia, and one in Sicily – would reduce tsunami detection time by more than 20 min for sources along the north Algerian and Tunisian shoreline. They also recommend the implementation of two tsunameters offshore at specific points in that area, to reduce the detection delay to less than 15–25 min along the Tunisian coast. However, the high installation and maintenance costs, along with the location of tsunamigenic areas so close to the coastline, has prevented the implementation of these offshore instruments in the M/BS area so far.
Since 2018, the Tsunami Last Mile project of the JRC explored how to better warn the population during near-shore or distant tsunami events. It was demonstrated twice – in Kos (autumn 2019; also presented at the AGU Fall Meeting in 2019) and in Malta (beginning of November 2021) – how interconnecting sensing devices with an alerting system may warn the population promptly and allow a safe evacuation. Possibly, the exercise will be repeated in Indonesia during 2022. The two events differ because Kos was a near-shore event and thus required the activation before the official alert from the service provider was issued, while Malta was a distant shore event so that the first activation was triggered by the information coming from the tsunami service providers. The system is based on a network including IDSLs and seismometers as sensing devices and a long-range siren with two alerting panels to warn the population. As soon as both IDSLs confirmed the anomalous behaviour of the sea level, the alerting devices started alerting the population automatically, providing indications about the countermeasures to be taken. In the case of Kos this was the first triggering of information. Specific signage deployed in the streets provided indications about the evacuation routes and the assembly points.
The system allows several degrees of automation, from completely automatic to manual activations only, depending on the standard procedures adopted by the authorities responsible for protecting the population. This system is based on the multi-purpose platform developed by the JRC and called RIO (Remote InterOperability), which is a complete software platform that allows easy integration of various instruments and analytical computations and activations. The RIO system was also adopted in another project, based on low-cost GNSS-based buoys that provide sea level data from the open sea. Such a type of device could be therefore naturally integrated in the same network and would provide the alerting information much in advance compared with devices deployed on the coasts: depending on the installation site, the improvement in reaction time could be considerable.
Traditionally, tide gauge stations have not been located in meteotsunami-prone areas but rather in locations of interest for other sea level processes (e.g. storm surges and sea level rise) and in large ports and harbours, where sea level data are of the utmost importance for the safety of navigation, or in the densely inhabited coastal towns. Up to the beginning of the 21th century, at meteotsunami hot spots, the measurements were done only during specific experiments, aimed purely at a better understanding of meteotsunamis and other high-frequency sea level phenomena. Such experiments include, but are not limited to, (1) several field experiments with simultaneous air pressure and sea level measurements done during the years 1989–1992 (Monserrat et al., 1991; Rabinovich and Monserrat, 1996) and 1996–1998 (Monserrat et al., 1998; Šepić et al., 2009) in Ciutadella (the Balearic Islands, Spain); (2) field experiments, during which bottom pressure was measured at several locations within the endangered Adriatic bays, conducted throughout the years 2007–2008 (Orlić and Pasarić, 2008) and 2015 (not published); (3) a field experiment with simultaneous air pressure and sea level measurements done in the year 2007 in Mazara del Vallo (Marrobbio Project Report, 2007; Zemunik et al., 2021a). Throughout the last 2 decades the national agencies and local authorities have, however, recognized a meteotsunami threat and have been making a continuous effort towards operational monitoring and forecasting of meteotsunamis, including the installation of permanent tide gauge stations at the most endangered coastal locations. These include Ciutadella (Menorca Island, Spain), where a tide gauge station has been operational since 2013, and Vela Luka, Mali Lošinj, and Stari Grad (the Adriatic Sea, Croatia), where permanent tide gauge stations were installed during 2017–2021. Having operational stations at meteotsunami-prone locations is a prerequisite for proper monitoring of destructive events, but it is not sufficient to implement an efficient warning system, given that a warning should be issued at least 1 h before the meteotsunami occurs at an endangered location.
Vilibić et al. (2016) presented a design of a meteotsunami warning system based on simultaneous numerical modelling of synoptic, mesoscale atmospheric and barotropic sea level conditions as well as a real-time assessment of atmospheric and ocean data measured a few tens up to a couple of hundred kilometres away from the most endangered spots, which is in locations where offshore meteotsunami generation and growth occur. Denamiel et al. (2019b, 2021) further upgraded the concept suggesting that stochastic approaches are more reliable than deterministic numerical modelling; the prototype of such a system, which gives estimates of the sea level exceedance probability during meteotsunamis, was tested for the Croatian coast of the Adriatic Sea (Denamiel et al., 2019b). Similar modelling and data assessment strategies have been suggested and tested for the Balearic Islands area (Renault et al., 2011; Šepić et al., 2016a; Romero et al., 2019), where a continuously upgraded meteotsunami warning system, with both deterministic and probabilistic components, has been operational since 1985 (Jansà and Ramis, 2021).
In spite of the great efforts invested in the development of the meteotsunami warning systems, the results are still not satisfactory, resulting in a loss of trust in the early warning systems. The forecasts are known to be wrong, especially when it comes to estimating the strength and destructiveness of the event (Jansà and Ramis, 2021). The crucial problem is an intrinsic inability of the atmospheric models – related, among other things, to coarse resolutions and inadequate physical parameterization at the mesoscale – to reproduce the exact properties (spatial outreach and rate of air pressure change, speed, pressure, and wind spatial gradient) of the fast-changing atmospheric disturbances responsible for the meteotsunami generation (Belušić et al., 2007). Slight changes in any of these properties (e.g. reducing the rate of air pressure change, moving meteotsunamigenic disturbances off their pathways for a few tens of kilometres, changing the propagation speed) may change the modelled sea level response by an order of magnitude, in particular at the most endangered areas (Vilibić et al., 2008; Orlić et al., 2010; Orfila et al., 2011; Šepić et al., 2016a; Ličer et al., 2017; Mourre et al., 2021).
Nevertheless, a significant densification of the observation network, including the sea level stations, onshore and offshore meteorological stations, and offshore bottom pressure recorders would help in real-time monitoring of meteotsunamigenic atmospheric disturbances and ocean conditions and in calibrating operational numerical models and would likely result in more reliable early warning systems.
4.2 Climate-related applications
4.2.1 Long-term variability and sea level trends
The main source of information to assess the long-term sea level trends and their variability is the annual and monthly means provided by the PSMSL (see Sect. 3.1). Another important source of information when looking at long-term trends is the estimation of the VLM which can be found in the GLOSS SONEL Data Assembly Center (https://www.sonel.org/, last access: 19 April 2022). To assess the availability of data for the M/BS in the PSMSL RLR dataset, we have made an extraction of the relative sea level trend product (https://www.psmsl.org/products/trends/, last access: 19 April 2022). This product fits a model composed of linear trend, seasonal component, and noise on data which have at least 70 % of their annual mean for the given time span. In this section four different time spans were considered, all ending in 2019; the shortest one is 30 years – which is the minimum time span of the online PSMSL product and roughly corresponds to the availability of satellite altimetry – while the longest considered time span is 100 years. The number of stations per country spanning the last 30, 50, 70, and 100 years is provided in Table 1.
Table 1Number of stations per country available for the different time spans of 30, 50, 70, and 100 years in the PSMSL sea level trend online product.

As coming from Table 1, the M/BS has very few centennial tide gauge records. If we consider the records spanning the last 100 years, seven stations are available at the PSMSL: four in the Mediterranean (Trieste, Venice, Bakar, and Marseille) and three in the Black Sea (Poti, Tuapse, and Sevastopol). Some stations that appear in the PSMSL database for the 1920–2019 period are not present in the shorter time spans period because they stopped in the second half of the 20th century. This is the case for Sevastopol that had 97 % of its annual record for the period 1910 to 1994 and then stopped, so it does not show up anymore in our 70, 50, and 30-year time spans.
For the sea level records spanning the last 70 years (1950–2019), 14 stations are available in the PSMSL data bank (2 times more than the centennial records). Four of these new long tide gauge records are located along the coast of Croatia (two are located in Split, one in Dubrovnik, and one in Rovinj) making the Adriatic Sea the most populated in terms of historical sea level records with half of the fourteen 70-year-long records. For Italy, France, and the Black Sea, the 70- and 100-year-long stations remain the same (except for Sevastopol discussed above), while Spain comprises three long records at the entrance of the Mediterranean Sea near the Strait of Gibraltar (Ceuta, Málaga, and Tarifa). The Alexandria station in Egypt also fits to the 70-year time span but disappears in the shorter time span because it ended in 2006 in the PSMSL database. It is noticeable that Alexandria is present up to 2016 in the UHSLC and that five of the new stations in this 70-year-long time span have not been updated in the PSMSL since 2017, highlighting the importance of updating records within the PSMSL. Although they do not appear yet in the PSMSL RLR dataset, some long-term records can be found from external sources. This is the case for Alicante station in Spain that has been recently digitized by Marcos et al. (2021), the Venezia station in Italy that has been updated recently (Zanchettin et al., 2021) and the data from Koper in Slovenia that has been provided by the co-authors of this paper.
If the last 30 years (1990–2019) are considered, the situation is a bit better, with 27 stations available. It is noticeable that no long-term records are available for the south of the Mediterranean Sea (north African coast). These long-term records are the only means to compute a robust estimation of sea level rise.
As an example of application mainly based on the PSMSL and SONEL datasets, we have computed the relative and absolute sea level trends in the stations where 70-year-long records are available (Fig. 9). We have decided to add also Alicante, Venezia, and Koper stations, not available in the PSMSL (represented in blue in Fig. 9). For Alicante I station, we have discarded for this trend computation historical data before 1910, due to a possible datum jump found in Marcos et al. (2021).
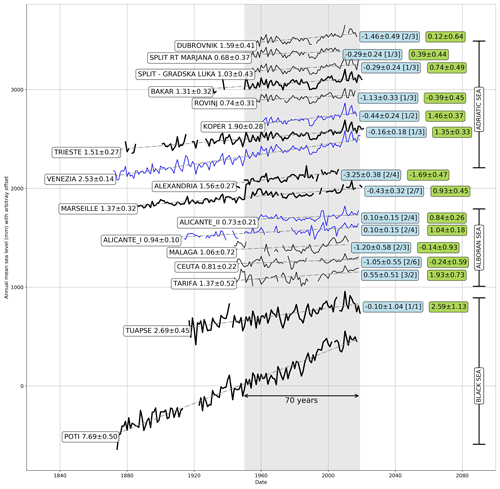
Figure 9Mean sea level evolution of the 70-year-long sea level records. The curves in black are for data from the PSMSL, those bold are the 100-year-long records, and those in blue the updated or new records, not available in the PSMSL. The name of the station is followed by the relative sea level trend; in the light blue boxes on the right of the curve is indicated the rate of VLM computed from different collocated GNSS stations and different solutions. The number in brackets indicates the number of GNSS stations/the number of solutions (i.e. [] means two collocated GNSS and seven different solutions) considered in the computation of the VLM estimates. The green boxes indicate the rate of absolute sea level change computed from the addition of relative sea level trend and vertical land motion. All estimates are in millimetres per year.
In Fig. 9 the vertical land movement (VLM) rates for each station are estimated from a combination of the different GNSS solutions provided by SONEL. These estimates are based on an aggregation of the different VLM solutions from the collocated GNSS stations, and they have been supported by an update on collocation between GNSS and tide gauges in Europe. The update has been recently released by the EuroGOOS Tide Gauge Task Team and SONEL, in the framework of a contract of EuroGOOS with the European Environmental Agency, to increase in situ sea level data provision to the Copernicus Marine Service (https://insitu.copernicus.eu/library/reports/coins-coastal-sealevel-stations, last access: 19 April 2022). It can be seen that all long-term records in the M/BS show relative sea level rise with values ranging from 0.68 mm yr−1 in Split Rt Marjana in the Adriatic Sea to 7.69 mm yr−1 in Poti in the Black Sea. The high sea level rise at this last station suggests that it is probably prone to significant VLM (Avsar et al., 2017). One can see also the high correlation of the sea level variation in the Adriatic Sea.
4.2.2 Evolution of extreme sea levels
Long-term changes, at interannual and longer timescales, in extreme sea levels are primarily driven by changes in mean sea level (MSL; Woodworth et al., 2019). However, variations in extremes unrelated to MSL variability have also been identified in tide gauge records at an hourly scale worldwide (Wahl and Chambers, 2015; Marcos and Woodworth, 2017) and linked to changes in storminess. In the Mediterranean Sea, long-term multi-decadal fluctuations in sea level extremes have been noticed in long tide gauge records, such as those in Trieste since the 1930s (Raicich, 2003) and other stations in the northern Adriatic (Masina and Lamberti, 2013), and in Marseille since the early 20th century (Letetrel et al., 2010; Marcos et al., 2015). These variations are regionally coherent as they normally originate from large-scale atmospheric forcing (Calafat et al., 2014; Marcos et al., 2015; Lionello et al., 2021). Given the variability at such low frequencies, the estimation of linear trends is highly dependent on the selected period. For example, Raicich (2003) identified a decrease in the frequency of extremes in the Trieste tide gauge record during the period 1940–2000, with no clear trend in their intensity. In contrast, Masina and Lamberti (2013) identified a small increase in the magnitude of sea level extremes in the northern Adriatic that was associated with an intensification of the bora wind in the 1990s. In Venice, Lionello et al. (2021) reported that the frequency of flooding resulting from storm surges has increased since the mid-20th century, although they linked this effect to relative sea level rise rather than to a sustained trend in storminess. As extremes are rare events, the detection of changes requires long time series to be reliable. Therefore, only a small subset of currently available tide gauge records is suitable for the identification of long-term changes in extreme sea levels. There is a geographical bias in the high-frequency long tide gauge records, with all time series over 50 years being located in the Western Mediterranean, with the only exception of Alexandria, and none in the Black Sea (Fig. 8). The investigation of changes in extreme sea levels is possible in the above-mentioned historical records that have been used in earlier studies. The location of these historical stations was chosen on the basis of criteria unconnected to the investigation of extreme episodes; one important example is the tide gauge in Marseille, which was initially devised to define the origin of the French national height system using accurate and continuous mean sea level measurements (Wöppelmann et al., 2014). Therefore, these stations are not necessarily placed in regions prone to the occurrence of extreme events. At interannual timescales changes in extreme sea levels are correlated with the North Atlantic Oscillation (Marcos et al., 2009b; Masina and Lamberti, 2013), even after the removal of the yearly MSL signal.
4.3 Satellite altimetry calibration
Nowadays satellite altimetry is an essential tool for mapping sea level changes over the ocean basins (Ablain et al., 2017). However, these measurements are biased by a number of processes (Fu and Haines, 2013), and therefore a proper calibration of these data is a prerequisite (Andersen and Cheng, 2013; Woodworth et al., 2017), in particular when approaching the coastal zone (Vignudelli et al., 2019). Further, there are multiple potential errors that may affect sea level estimates, in particular close to the coast. These include spurious trends in the geophysical corrections, an imperfect inter-mission bias estimate, a decrease in valid data close to the coast, and errors in waveform retracking (Bosch et al., 2014). The tide gauge data are normally used for satellite altimetry calibration, yet the problem is that the altimetry measurements are not reliable close to land, while large differences in mean sea level may occur at coastal distances (e.g. in the last 4–5 km to the coast, as observed for the Senetosa calibration site in Corsica for both TOPEX/Poseidon and Jason altimetry missions, Gouzenes et al., 2020). Here, for the Senetosa calibration site, it has been proven that the steric sea level component is responsible for such changes (Dieng et al., 2021).
These problems are even more amplified in enclosed seas, such as the M/BS, where the impact of a basin topography and a complex coastline is more pronounced than in the oceans. Orbit-related sea level errors are an example, found to be prominent in the Mediterranean (Esselborn et al., 2020). For that reason, the calibration of satellite altimeters has a long history there. This particularly applies to some selected tide gauge sites at the Mediterranean locations, like Ibiza (Martinez-Benjamin et al., 2004; Frappart et al., 2015) or Corsica (Bonnefond et al., 2003, 2021; Cancet et al., 2013). Recently, advanced learning methods (such as deep-learning networks) have been used to improve calibrations of altimeter data (Yang et al., 2021).
To conclude, a very limited number of tide gauges is placed in the M/BS at locations both directed towards the open sea and being in the track of a satellite, while there is a clear lack of open-ocean buoys with a tide gauge and a GNSS receiver capable of precise positioning (Quarly et al., 2021). Indeed, such systems may minimize the errors in satellite altimetry calibrations, while a state-of-the-art GNSS-based technological solution may also be deployed for improving estimates at coastal calibration sites (Bonnefond et al., 2022).
4.4 Definition of vertical frames of reference
The precise information on vertical references, which strongly relies on tide gauge measurements, is also a major issue, for both ocean and land charts. Vertical reference surfaces can be categorized under three general headings:
-
a tidal datum, also called a chart datum, which should, according to the IHO recommendations, correspond to the lowest astronomical tide (LAT) or an equivalent reference level regarded by the hydrographic services as being as close as possible to the LAT;
-
a vertical reference datum or a geodetic datum, which is a surface of zero elevation to which heights of various points at land are referred, being a base for defining height systems at a national level (national geodetic datum) or at a regional scale (e.g.: the European Vertical Reference System, EVRF2019);
-
an ellipsoidal reference datum, a global geodetic datum which allows us to define sea level heights relative to a reference ellipsoid (mathematically defined earth surface that approximates the geoid or equipotential surface). The ellipsoidal height is important for satellite altimetry measurements and GNSS receivers at tide gauges (e.g. Adebisi et al., 2021), such as the Global Reference System 1980 (GRS80) or the World Geodetic System 1984 (WGS84).
At tide gauges, the relationship between these three data must be known to allow the observational data to serve all possible applications (e.g. the analysis of the tidal observations allows us to define the characteristics of the tide at the tide gauges, such as the lowest and highest levels and MSL, and consequently to determine the chart datum).
Traditionally, bathymetric data have been collected and stored relative to the chart datum and topographic data relative to a geodetic datum. Close to a tide gauge, bathymetric data can be referenced to the chart datum by subtracting the observations of the tide gauge directly or in association with models. One of the most significant challenges in traditional hydrography is establishing the relationship between the instantaneous water surface and the chart datum away from water level gauge locations. In this case, to obtain the chart depths, the vertical positions of the bottom are referenced to the so-called vertical reference surface for hydrography (VRSH), which, following the recommendations of the IHO, is identified with the development of a 3D separation model between the chart datum and the geodetic datum (geoid and/or with respect to the ellipsoid).
In modern times, the hydrographic surveying community uses high-accuracy GNSS positioning techniques for vertical positioning of survey platforms, the sea surface and the sea floor. This method of hydrographic surveying, which is known as ellipsoidal referenced surveying (Hamden and Din, 2018), provides a direct measurement of the seafloor to the ellipsoid, as established by GNSS observations, and a translation of the reference from the ellipsoid to the geoid and/or a chart datum (VRSH).
As an example, in Spain the orthometric and ellipsoidal heights of the chart datum have been established for the Iberian Peninsula and the Canary Islands domains from model-reanalysis sea level data fields, which were first validated and then adjusted to experimental data from 119 tide gauge stations. The sea level height with respect to the geoid was obtained from the modelling-reanalysis service provided by the Iberia–Biscay–Ireland Monitoring and Forecasting Center (IBI MFC), in the framework of the EU Copernicus Marine Environment Monitoring System (Sotillo et al., 2015). Away from water level gauge locations the model will be adjusted in the future by GNSS water level buoys, to establish the chart datum at offshore locations.
An overview of existing coastal sea level infrastructure in the M/BS has been presented, based on the contribution from 30 institutions/sea level scientists operating tide gauges in the region. These stations are essential to monitor and study sea level variations that pose a hazard in these basins, such as those produced by storm surges, tsunamis, meteotsunamis, and sea level rise, by providing accurate sea level data at all frequency ranges along the coastline. The initiative gives an insight into the status of the in situ sea level network in both basins and confirms several challenging aspects such as the diversity of national strategies, sea level technology, funding, or data availability, often linked to differences in the primary and evolving objectives of these installations and their unbalanced spatial distribution. National contacts and relevant basic metadata are provided, as a starting point for improving coordination across the region. In most countries tide gauges are operated by several agencies, usually targeting different purposes. Only seven of these institutions are in charge of more than 15 stations, while the majority operate a smaller number of stations and sometimes one single station.
We have identified 240 active stations covering nearly all the country's coastlines in the M/BS. Several stations in Morocco and Tunisia could not be added to this inventory, and no information was obtained from Libya, confirming the lack of information along the southern Mediterranean coast from previous initiatives.
There are still many float gauges such as the ones installed since the end of the 19th century for tides and hydrography, still the second most important application, especially along the northern and eastern Adriatic and the Greek coasts. However, tides in the M/BS are generally small, so tide predictions are not computed nor needed for port operations everywhere. Therefore, an increasing number of stations are becoming multi-purpose, a term we apply here to tide gauges upgraded to be used also in tsunami, storm surge and other early warning systems. A significant part of the network is based on radar sensors (134; 56 % of the stations) providing 1 min time sampling data, with real-time or near-real-time data transmission. This has been mainly driven by new requirements for tsunami warning systems since 2005, which yielded to the upgrade of existing networks in several countries (e.g. Spain, France, Italy, Türkiye) and the installation of new sensors in several areas (15 new radar sensors since 2012 along the Greek coast (NOA), 24 inexpensive acoustic sensors by the JRC, in collaboration with several national operators, all around the Mediterranean). In fact, about 152 of the active stations nowadays contribute to regional or national tsunami warning systems in this region. In addition, as for the global network, lower time samplings have allowed an improved dataset for understanding and warning of meteotsunami events, more frequent than tsunamis at several spots in the M/BS. While 1 min sampling is sufficient for the detection of most of these sea level oscillations, access to even higher-frequency raw data from modern sensors would be desirable in the future, for a better characterization of all periods above 30 s. As an example, PdE in Spain has recently developed a tool for the operational characterization of 2 Hz raw data from the REDMAR network, which provides these data and derived data products including waves through the PdE OPeNDAP server. In addition, at least four more institutions participating in this survey compute wind wave parameters from tide gauge raw data.
Focussing on long sea level records, numbers decrease significantly: only 10 stations have been identified with enough valid data covering the last 100 years (7 with data in the PSMSL), while 27 would have data for the last 30 years (the altimetry period). This reflects the limitations of the network to provide reliable sea level trends and their spatial variability along the coastline. This is perhaps the most challenging application, not only because of the smaller number of tide gauges in the past but also since it requires a precise knowledge of the station history, data archaeology efforts, and often the careful combination of data from different technologies/locations inside a harbour. Fortunately, access to VLM information has also improved in recent years, with up to 46 stations now collocated with a permanent GNSS in the M/BS.
Some of these stations are contributing, with different time sampling, latency, and quality control, to one or more of the seven international data integrators or portals described in Sect. 3, where a detailed assessment of data accessibility in the M/BS is presented. The most populated portal, the PSMSL, contains data from 158 stations in the region, which means that there is still a significant number of M/BS stations focused on local or national services and not included in international programmes. This is a problem for basin-scale applications and research studies, and it is often related to data policy issues: not all tide gauges provide open and free data to users, and some claim data availability only for research applications. It must be emphasized that most national operators rely on their own personnel and resources, including in situ maintenance as well as quality control, data processing, and product generation, and international programmes have traditionally relied on these national efforts. This requires enough funding of the networks from the Member States, which is not always guaranteed, and it could also explain the lack of access to data or the delays in updating the time series with recent records.
By contrast, in other cases, the same sea level time series can be found in different repositories as well as in national data portals, with a different name, metadata, or quality control, which may be confusing for end users. These problems are not exclusive to the M/BS, and are partly linked to the lack of unique identifiers and adequate and standard metadata information for tide gauges. Data integrators should collaborate between them and work more closely with original data providers, to ensure interoperability and homogeneous and good-quality datasets, according to FAIR principles. These issues are being tackled by GLOSS and by the EuroGOOS Tide Gauge Task Team in the framework of the EuroSea project (see EuroSea Deliverable 3.3: New tide gauge data flow strategy, https://oceanrep.geomar.de/id/eprint/52175/, last access: 18 June 2022).
This work shows the evolution of sea level sensor technology through the decades, for tide gauges typically installed on a pier in a harbour. These are relatively easy to install and maintain and the main source of in situ sea level data along the coast. Apart from float, acoustic, pressure, and radar sensors, a novel technique based on GNSS interferometric reflectometry (GNSS-IR) has recently emerged and revealed its potential as the number of satellite constellations has increased (Peng et al., 2021). GNSS receivers have the advantage of providing both sea level and land motion information. Some institutions in the M/BS (e.g. Spanish Geographic Institute) are already exploring this technique. In the framework of the EuroSea project and the EuroGOOS Tide Gauge Task Team activities, the UK National Oceanographic Center (NOC) has recently developed a global GNSS-IR data portal, hosted at the PSMSL (https://eurosea.eu/new/a-global-sea-level-data-portal-using-global-navigation-satellite-system-interferometric-reflectometry/, last access: 18 June 2022).
The use of GNSS receivers for sea level is becoming a reality, and it is going even further. For many applications, in situ sea level measurements offshore would be a significant improvement of the coastal sea level network. One example is tsunami warning, where the detection of the wave before reaching the coast is a clear advantage. GNSS receivers on buoys (GNSS buoys) have been used for years in Japan's tsunami warning system. Despite the fact that this inventory does not include these types of stations in the M/BS, we know that several countries and agencies are now planning their implementation by adding GNSS receivers to existing or new buoys. These data would be also valuable for the calibration of coastal altimetry or, as described in Sect. 2, for the determination of the offshore chart datum.
The present review has also revealed that at least 64 % of active stations in the M/BS (150) have some kind of ancillary sensor providing meteorological and/or oceanographic data. Atmospheric pressure and wind are the most traditional and frequent additional parameters, very often with time samplings of 1 min, useful for meteotsunami studies. But a number of stations in the Appendix are in fact multi-sensor platforms providing a large range of parameters, as do meteorological stations (humidity, air temperature, precipitation, etc.), and even ocean data like water temperature, salinity, or currents. Several agencies have already installed or plan to install cameras (e.g. webcams planned by SHOM, in France). Multi-sensor platform deployment seems a reasonable approach for ensuring sustainability of the networks by expanding their range of applications even more.
In summary, the present assessment of the coastal sea level monitoring capacities in the M/BS exposed several important issues that could be improved for making sea level operations and science over a variety of timescales and applications in these enclosed basins comprehensive: (1) the longevity of calibrated measurements is threatened at some monitoring sites, which may lower the confidence of the sea level rise estimates in the era of climate change – this should be immediately bypassed by again putting into operation all inoperable tide gauges that have multi-decadal time series; (2) the spatial gaps in monitoring systems (or their inadequacy) that exist on some coastlines (e.g. Libya, Albania) should be bridged, by upgrading the existing stations or installing new ones; (3) the quoted activities should be done through collaboration and knowledge transfer from more experienced tide gauge networks towards less experienced ones (e.g. northern African countries), preferably within the umbrella of existing international programmes (IOC, MONGOOS), agencies (e.g. through extending the IDSL network of the JRC), or joint projects; (4) the data should be available for research and follow the open-science policies, in particular the FAIR principles (Wilkinson et al., 2016); and (5) the multitude of sea level data repositories should be minimized, with the clear and unique provision of data for real-time and research purposes through a one-stop shop service, including harmonized quality-check procedures. We hope that the next decade of coastal sea level monitoring will be as dynamic as the last decade, in which substantial progress in some of the quoted issues has been achieved in some tide gauge networks, thus with a potential to be extended over the whole Mediterranean and Black seas.
Table A1Stations operated by Puertos del Estado, Spain (REDMAR network) and data availability. Data available at https://portus.puertos.es/?locale=en#/ (last access date: 18 June 2022).
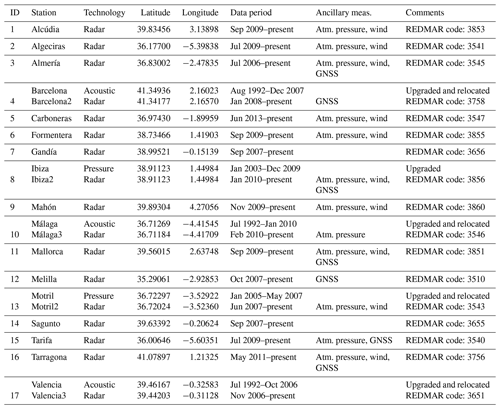
Table A3Stations operated by National Geographic Institute of Spain in the Mediterranean Sea and data availability.
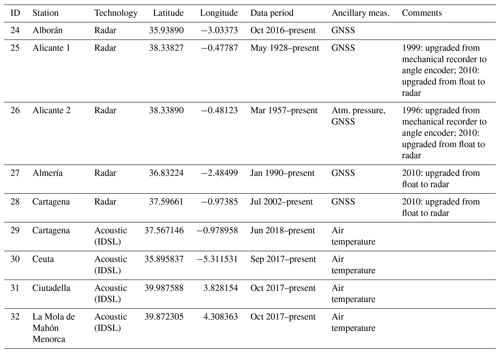
Table A4Stations operated by Spanish Institute of Oceanography in the Mediterranean Sea and data availability.
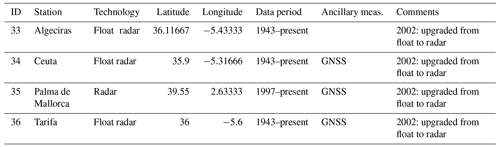
Table A5Stations operated by Spanish Hydrographic Office in the Mediterranean Sea and data availability.
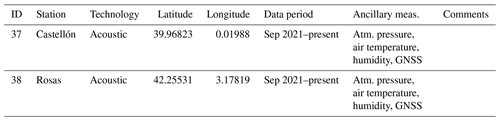
Table A6Station operated by Josep Pascual (meteorological observer, Meteolestartit/CSIC) in Catalonia (Spain).

Table A7Stations operated by SHOM (RONIM network) in France. Data available at https://data.shom.fr/donnees/refmar/nnn, last access: 11 July 2022 (nnn: station code in SHOM).
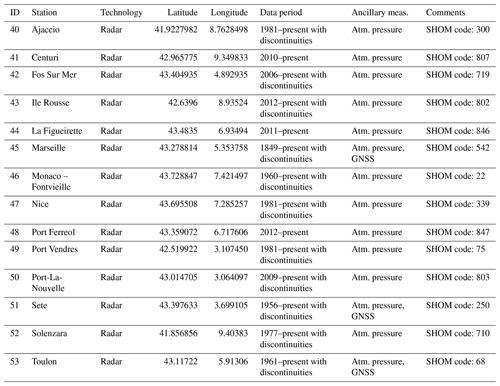
Table A8Stations operated by ISPRA – Italian Institute for Environmental Protection and Research (RMN – national tide gauge network; RMLV – Venice Lagoon and North Adriatic Tide Gauge Network).
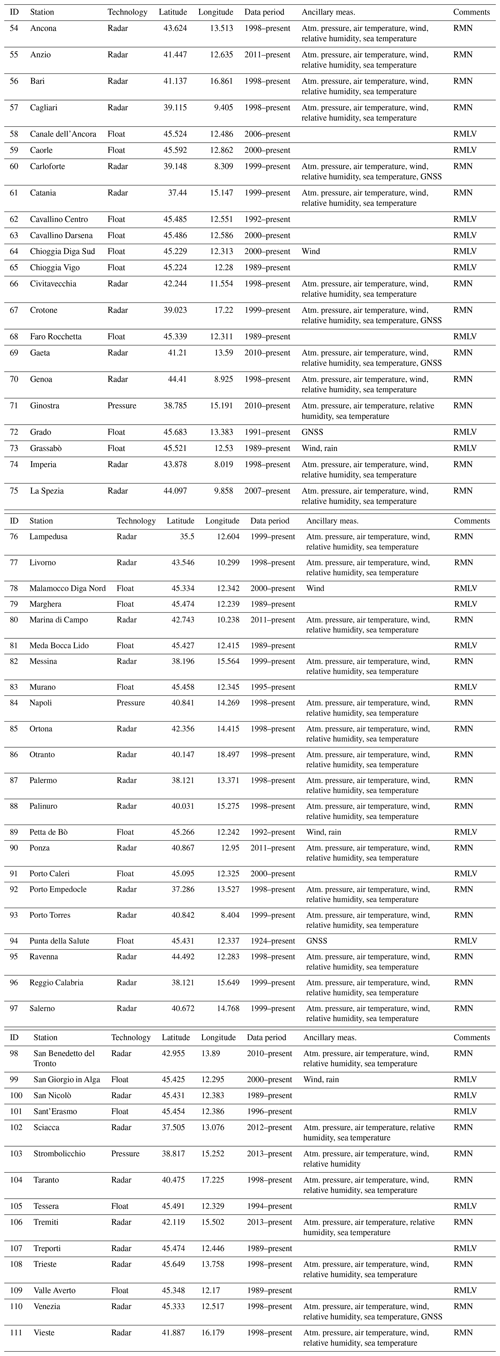
Table A11Slovenian tide gauge station operated by the Slovenian Environment Agency. Data available at http://www.arso.gov.si/vode/podatki/amp/H9350_g_1.html (last access: 18 June 2022).

Table A12Croatian tide gauge stations operated by the Andrija Mohorovičić Geophysical Institute (AMGI), Hrvatske Vode (HV), Hydrographic Institute of the Republic of Croatia (HHI), Institute of Oceanography and Fisheries (IOF), and Ruđer Bošković Institute (RBI).
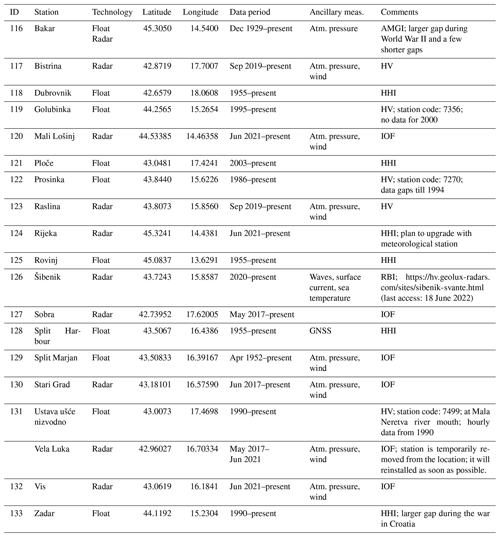
Table A13National tide gauge network of Montenegro operated by the Institute for Hydrometeorology and Seismology.

Table A20North and north-eastern Black Sea tide gauge stations operated by the All-Russian Research Institute of Hydrometeorological Information – World Data Center.
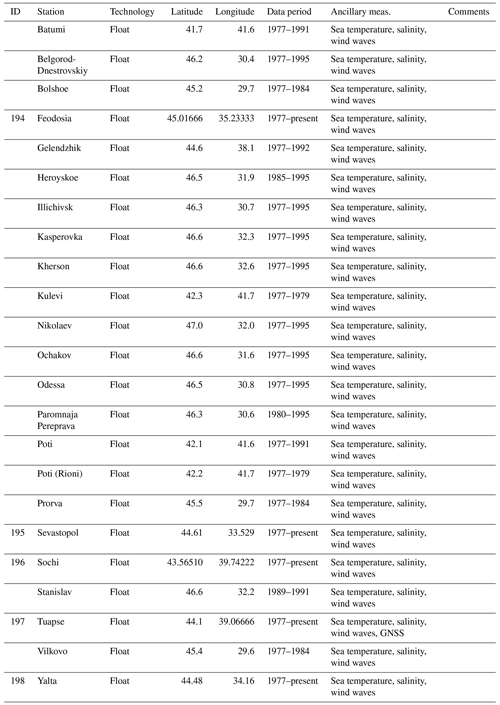
Table A21Turkish Sea Level Monitoring System (TUDES) tide gauge stations operated by the General Directorate of Mapping and data availability.
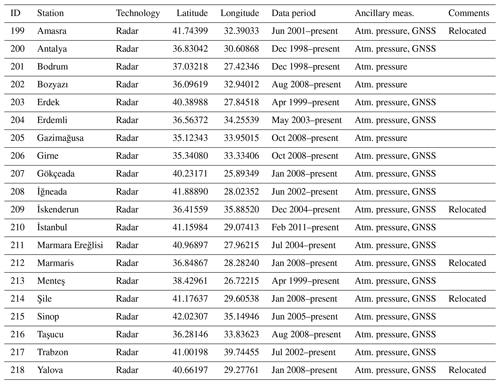
Table A23Cyprus sea level/tide gauge stations deployed/operated by the DFMR, OC-UCY, DLS, CUT, JRC, and ORION.
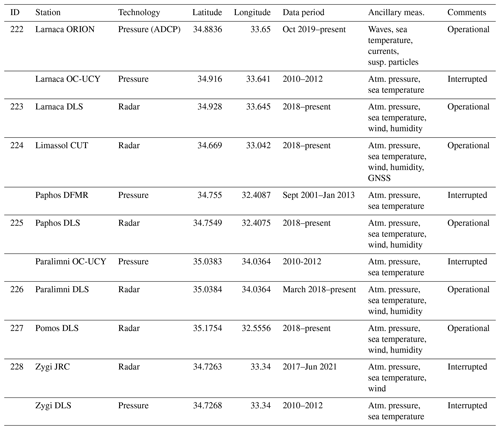
Table A24Tide gauge stations in Israel, operated by the National Institute of Oceanography, Israel Oceanographic and Limnological Research.
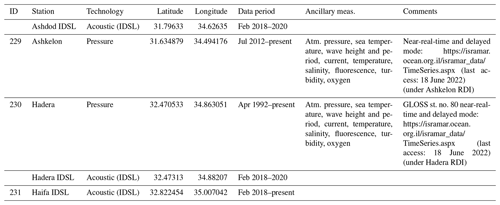
List of abbreviations | |
ADCP | Acoustic Doppler current profiler |
AMGI | Andrija Mohorovičić Geophysical Institute (Croatia) |
BMA | Bayesian model average |
BOLAM | Hydrostatic Meteorological Limited Area Model developed by CNR-ISAC (Italian National Research Council, Institute of Atmospheric Sciences and Climate, Bologna) |
CA | Cadastre Agency, Ministry of Regional Development and Public Works) |
CENALT | Centre d'alerte aux tsunamis, French Tsunami Warning Centre |
CIESM | The Mediterranean Science Commission, Intergovernmental Commission for the Scientific Exploration of the Mediterranean Sea |
CHANGE WE CARE | Climate cHallenges on coAstal and traNsitional chanGing arEas: WEaving a Cross-Adriatic REsponse; RESPONSe, Strategies to adapt to climate change in Adriatic regions |
CNR | Italian National Research Council |
CNR-ISAC | Italian National Research Council, Institute of Atmospheric Sciences and Climate, Bologna |
CNR-ISMAR | CNR Institute of Marine Sciences |
CUT | Cyprus University of Technology |
DFMR | Department of Fisheries and Marine Research Oceanography Unit (Cyprus) |
DLS | Department of Lands and Surveys in Cyprus |
ECMWF | European Centre for Medium-Range Weather Forecasts |
EMODnet | European Marine Observation and Data Network, https://emodnet.ec.europa.eu/en (last access: 18 June 2022) |
ENSURF | Ensemble SURge Forecast |
ESEAS-RI | European Sea Level Service Research Infrastructure (European Framework Programme 5) |
EVRF2019 | European Vertical Reference System 2019 |
FAIR | Findable, accessible, interoperable, and reusable |
GESLA | Global Extreme Sea Level Analysis, https://gesla787883612.wordpress.com/ (last access: 18 June 2022) |
GLOSS | Global Sea Level Observing System |
GNSS | Global Navigation Satellite System |
GNSS-IR | GNSS interferometric reflectometry |
GPRS | General Packet Radio Service (packet oriented mobile data standard on 2G/3G/4G for mobile communications) |
GRS80 | Global Reference System 1980 |
GSM | Global System for Mobile Communications |
HHI | Hydrographic Institute of the Republic of Croatia |
HNHS | Hellenic Navy Hydrographic Service |
HV | Hrvatske vode (Croatian Waters) |
IBI-MFC | Iberia–Biscay–Ireland Monitoring and Forecasting Centre (Copernicus Marine Service) |
ICG/NEAMTWS | Intergovernmental Coordination Group for the North-Eastern Atlantic, the Mediterranean and Connected Seas Tsunami Warning and Mitigation System |
ICM/CSIC | Instituto de Ciencias del Mar/Consejo Superior de Investigaciones Científicas (Marine Sciences Research Institute in Barcelona) |
IDSL | Inexpensive Device for Sea Level |
IEO | Instituto Español de Oceanografía (Spanish Oceanographic Institute) |
IGN | Instituto Geográfico Nacional (National Geographic Institute of Spain) |
IHM | Instituto Hidrográfico de la Marina (Spanish Hydrographic Office) |
IHO | International Hydrographic Organization |
INCT | National Institute of Cartography and Remote Sensing Algeria |
Interreg | Series of programmes to stimulate cooperation between regions in and out of the European Union (EU), funded by the European Regional Development Fund: oceanographic observations for biodiversity |
IO-BAS | Institute of Oceanology, Bulgarian Academy of Sciences (IO-BAS) |
IOC/UNESCO | Intergovernmental Oceanographic Commission from UNESCO |
IOC-SLSMF | Intergovernmental Oceanographic Commission Sea Level Station Monitoring Facility |
IOF | Institute of Oceanography and Fisheries in Croatia |
IOLR | Israel Oceanographic and Limnological Research |
ISMAR | Institute of Marine Sciences (Italy) |
ISPRA | Istituto Superiore per la Protezione e la Ricerca Ambientale (Italian Institute for Environmental Protection and Research, Italy) |
JRC | Joint Research Centre, European Union |
JRC-SLD | Joint Research Centre sea level database |
JRC-TAD | Joint Research Centre Tsunami Alert Device |
KOERI | Kandilli Observatory and Earthquake Research Institute |
LAT | Lowest astronomical tide |
M/BS | Mediterranean and Black Sea |
MedGLOSS | Mediterranean component of the Global Sea Level Observing System |
MED-MFC | Mediterranean Monitoring and Forecasting Centre (Copernicus Marine Service) |
MESSI | Meteotsunamis, destructive long ocean waves in the tsunami frequency band: from observations and simulations towards a warning system |
MISELA | Minute Sea Level Analysis product |
MONGOOS | Mediterranean Oceanography Network for the Global Ocean Observing System, http://www.mongoos.eu/ (last access: 18 June 2022) |
MSL | Mean sea level |
NAO | North Atlantic Oscillation |
NIMH | National Institute of Meteorology and Hydrology, Bulgarian Academy of Sciences |
NIMRD “Grigore Antipa” | National Institute for Marine Research and Development “Grigore Antipa” (Romania) |
NIOF | National Institute of Oceanography and Fisheries |
NOA | National Observatory of Athens |
NOC | National Oceanographic Centre (UK) |
PdE | Puertos del Estado (Ports of Spain) |
PI | Port Infrastructure (Bulgaria) |
POZOR | Monitoring of potentially dangerous sea level oscillations and their contribution to floods of coastal areas in the future climate |
PSMSL | Permanent Service for Mean Sea Level |
OC-UCY | Oceanography Centre University of Cyprus |
OPeNDAP | Open-source Project for a Network Data Access Protocol |
ORION | Joint Research and Development Centre, Cyprus |
RBI | Ruđer Bošković Institute |
REDMAR | Sea level observation network of Spanish Harbours |
REDNAP | Red de Nivelación de Alta Precisión (High Precision Levelling Network) |
RIO | Remote InterOperability |
RLR | Revised Local Reference |
RMLV | Rete Mareografica della Laguna di Venezia e del Litorale Nord Adriatico, Venice Lagoon and North Adriatic Tide Gauge Network |
RMN | Rete Mareografica Nazionale, Italian National tide gauge network |
RONIM | Sea level observation network in France |
SeaDataNet | Pan-European infrastructure for ocean and marine data management |
SHOM | Service Hydrographique et Oceanographique de la Marine (France), French Naval Hydrographic Oceanographic Service |
SHYFEM | Shallow Water Hydrodynamic Finite Element Model |
SOCIB | Balearic Islands Coastal Observing and Forecasting System |
SONEL | GNSS data assembly centre for the Global Sea Level Observing System (GLOSS) |
TAC | Thematic Assembly Centre |
TGBM | Tide gauge benchmark |
TOPEX/Poseidon | Topography Experiment/Poseidon |
TUDES | Turkish National Sea Level Monitoring System |
UHSLC | University of Hawaii Sea Level Center |
VLM | Vertical land movement |
VPN | Virtual private network |
VRSH | Vertical reference surface for hydrography |
WGS84 | World Geodetic System 1984 |
ZMPS | Zero tide level used as reference benchmark for tide measurements in the Lagoon of Venice |
Access to sea level data presented in this review is provided through national and international data portals and repositories quoted in Sects. 2 and 3 and in the Supplement.
The supplement related to this article is available online at: https://doi.org/10.5194/os-18-997-2022-supplement.
BPG led this work as chair of the MONGOOS Tide Gauge Task Team and was in charge of the overall direction and planning, based on discussions with team members (IV, JS, LT, FR, MM, CF, ML, SM, GZ) for definition of the final scope and structure of the paper. Several authors took the lead in writing specific sections: BPG (Sects. 1, 2 and 5), IV (Abstract, Sects. 3 and 4.3), CF (Sect. 4.1.1 and 4.4), ML (Sect. 4.1.2), JŠ (Sect. 4.1.3), LT (Sect. 4.2.1), and MM (Sect. 4.2.2). The following authors provided relevant contributions to Sect. 2, including network description, answers to the survey, and station information for their respective institutions in Spain (BPG, EAF, MM, JT, BC, ET, AC, VMG, MAF, JMQB, MLR, JP, and JS), France (CF), Italy (SM, MP, FR), Malta (AD, AG), Slovenia (MJ), Croatia (HM, SČ, DB, IM, JŠ, IV), Montenegro (BG), Albania (KZ), Greece (GS, DAG), Cyprus (GZ), Egypt (MS), Israel (AL), Türkiye (MS, ES, HK), Bulgaria (AP), Romania (DN), Russia (IM), Algeria (HA, AM, MAM), and those from the JRC, including the Lebanon IDSL station (DAG). BPG designed and launched the survey to identified contacts, prepared Figs. 2 and 3, and contributed to writing in Sect. 4.1.1, 4.1.2, 4.1.3, and 4.2.1. JŠ provided the meteotsunami description in Sect. 1, and prepared Fig. 1. Maximum hourly values for Fig. 1a were provided by MM (for those stations in the GESLA dataset), MS (stations in Türkiye), GS (Greek stations), and IM and SČ (Croatian stations). IM designed and prepared Figs. 4, 5, and 6 with detailed mapping of existing infrastructure, based on the station list in the Appendix, and IV and JŠ prepared Figs. 7 and 8 on data availability in data portals. LT compiled monthly mean sea levels from PSMSL and several agencies, and prepared Fig. 9. JMQB and MLR contributed to Sect. 4.1.1 and 4.4, SM and MP to Sect. 4.1.2, and DAG to Sect. 5. JŠ, SČ, DB, MJ, and ML helped with contacting authors from Algeria, Russia, Montenegro, and Albania. Finally, IV, JS, IM, and BPG helped shape the final version of the paper during the internal review process. All authors have read and agreed to the submission of the paper for publication.
The contact author has declared that neither they nor their co-authors have any competing interests.
Publisher’s note: Copernicus Publications remains neutral with regard to jurisdictional claims in published maps and institutional affiliations.
This article is part of the special issue “Advances in interdisciplinary studies at multiple scales in the Mediterranean Sea”. It is a result of the 8th MONGOOS Meeting & Workshop, Trieste, Italy, 3–5 December 2019.
This collaborative work has been possible thanks to the MONGOOS (Mediterranean Operational Network for the Global Ocean Observing System) network, aimed at long-term synergies in the Mediterranean Sea. It has been developed in the framework of the MONGOOS Tide Gauge Task Team, as a contribution to EuroGOOS Tide Gauge Task Team and GLOSS (Global Sea Level Observing System) main objectives and activities in the M/BS. The authors would like to express their gratitude to local and national technicians, harbour personnel, and experts that have been in charge of the continuous operation and maintenance of tide gauge networks in the region, most of the times through national funding (e.g. MASRI, Bulgaria) and others with the help of international programmes (IOC/UNESCO, CIESM, EU framework programmes, Interreg programmes), often facing severe difficulties to ensure permanent installations and sustainable networks. We also acknowledge the effort of data aggregators and portals (PSMSL, GLOSS, Copernicus Marine Service In Situ TAC, EMODnet, IOC-SLSFM, JRC-SLD, etc.) in managing harmonization and generating improved datasets for scientific research.
This research has been partially supported by Horizon 2020 (EuroSea (grant no. 862626) and JERICO-S3 (grant no. 871153)).
This paper was edited by Alejandro Orfila and reviewed by two anonymous referees.
Aarup, T., Wöppelmann, G., Woodworth, P. L., Hernandez, F., Vanhoorne, B., Schöne, T., and Thompson, P. R.: Comments on the article “Uncertainty and bias in electronic tide-gauge records: evidence from collocated sensors” by Stella Pytharouli, Spyros Chaikalis, Stathis C. Stiros in Measurement (Volume 125, September 2018), Measurement, 135, 613–616, https://doi.org/10.1016/j.measurement.2018.12.007, 2019.
Ablain, M., Legeais, J. F., Prandi, P., Marcos, M., Fenoglio-Marc, L., Dieng, H. B., Benveniste, J., and Cazenave, A.: Satellite altimetry-based sea level at global and regional scales, Surv. Geophys., 38, 7–31, https://doi.org/10.1007/s10712-016-9389-8, 2017.
Adebisi, N., Balogun, A. L., Min, T. H., and Tella, A.: Advances in estimating Sea Level Rise: A review of tide gauge, satellite altimetry and spatial data science approaches, Ocean Coast. Manag., 208, 105632, https://doi.org/10.1016/j.ocecoaman.2021.105632, 2021.
Alasset, P. J., Hébert, H., Maouche, S., Calbini, V., and Meghraoui, M.: The tsunami induced by the 2003 Zemmouri earthquake (MW = 6.9, Algeria): modelling and results, Geophys. J. Int., 166, 213–226, https://doi.org/10.1111/j.1365-246X.2006.02912.x, 2006.
Álvarez Fanjul, E., Pérez-Gómez, B., and Rodríguez, I.: Nivmar: a storm surge forecasting system for Spanish waters, Sci. Mar., 65, 145–154, https://doi.org/10.3989/scimar.2001.65s1145, 2001.
Álvarez-Gómez, J. A., Aniel-Quiroga, ĺ., González, M., and Otero, L.: Tsunami hazard at the Western Mediterranean Spanish coast from seismic sources, Nat. Hazards Earth Syst. Sci., 11, 227–240, https://doi.org/10.5194/nhess-11-227-2011, 2011.
Amato, A.: Some reflections on tsunami Early Warning Systems and their impact, with a look at the NEAMTWS, Boll. Geof. Teor. Appl., 61, 403–420, https://doi.org/10.4430/bgta0329, 2020.
Amores, A., Marcos, M., Carrió, D. S., and Gómez-Pujol, L.: Coastal impacts of Storm Gloria (January 2020) over the north-western Mediterranean, Nat. Hazards Earth Syst. Sci., 20, 1955–1968, https://doi.org/10.5194/nhess-20-1955-2020, 2020.
Andersen, O. B. and Cheng, Y.: Long term changes of altimeter range and geophysical corrections at altimetry calibration sites, Adv. Space Res., 51, 1468–1477, https://doi.org/10.1016/j.asr.2012.11.027, 2013.
Androulidakis, Y. S., Kombiadou, K. D., Makris, C. V., Baltikas, V. N., and Krestenitis, Y. N.: Storm surges in the Mediterranean Sea: variability and trends under future climatic conditions, Dyn. Atm. Oceans, 71, 56–82, https://doi.org/10.1016/j.dynatmoce.2015.06.001, 2015.
Avsar, N. B., Jin, S. G., Kutoglu, S. H., and Gurbuz, G.: Vertical land motion along the Black Sea coast from satellite altimetry, tide gauges and GPS, Adv. Space Res., 60, 2871–2881, https://doi.org/10.1016/j.asr.2017.08.012, 2017.
Bajo, M. and Umgiesser, G.: Storm surge forecast through a combination of dynamic and neural network models, Ocean Model., 33, 1–9, https://doi.org/10.1016/j.ocemod.2009.12.007, 2010.
Bajo, M., Međugorac, I., Umgiesser, G., and Orlić, M.: Storm surge and seiche modelling in the Adriatic Sea and the impact of data assimilation, Q. J. Roy. Meteorol. Soc., 145, 2070–2084, https://doi.org/10.1002/qj.3544, 2019.
Banks, A., Drakopoulos, P., and Zodiatis, G.: A comparison of tide gauge and satellite altimetry derived sea-level for two eastern Mediterranean islands, Geophys. Res. Abstracts, 5, European Geophysical Society – American Geophysical Union – European Union of Geophysicists Joint Assembly, Nice, France, https://ui.adsabs.harvard.edu/abs/2003EAEJA....13157B/abstract (last access: 11 July 2022), 6–11 April 2003.
Beltrami, G. M., Di Risio, M., and De Girolamo, P.: Algorithms for automatic, real-time tsunami detection in sea level measurements, in: The Tsunami Threat – Research and Technology, edited by: Morner, N.-A., InTech Open, 549–574, https://doi.org/10.5772/13908, 2011.
Belušić, D., Grisogono, B., and Klaić, Z. B.: Atmospheric origin of the devastating coupled air–sea event in the east Adriatic, J. Geophys. Res., 112, D17111, https://doi.org/10.1029/2006JD008204, 2007.
Bernier, N. B. and Thompson, K. R.: Deterministic and ensemble storm surge prediction for Atlantic Canada with lead times of hours to ten days, Ocean Model., 86, 114–127, https://doi.org/10.1016/j.ocemod.2014.12.002, 2015.
Bertotti, L., Bidlot, J.-R., Buizza, R., Cavaleri, L., and Janousek, M.: Deterministic and ensemble-based prediction of Adriatic Sea sirocco storms leading to “acqua alta” in Venice, Q. J. Roy. Meteor. Soc., 137, 1446–1466, https://doi.org/10.1002/qj.861, 2011.
Bevacqua, E., Maraun, D., Vousdoukas, M. I., Voukouvalas, E., Vrac, M., Mentaschi, L., and Widmann, M.: Higher probability of compound flooding from precipitation and storm surge in Europe under anthropogenic climate change, Sci. Adv., 5, eaaw5531, https://doi.org/10.1126/sciadv.aaw5531, 2019.
Bitharis, S., Ampatzidis, D., Pikridas, C., Fotiou, A., Rossikoppoulos, D., and Schuh, H.: The role of GNSS vertical velocities to correct estimates of sea level rise from tide gauge measurements in Greece, Mar. Geodesy, 40, 297–314, https://doi.org/10.1080/01490419.2017.1322646, 2017.
Bonaduce, A., Pinardi, N., Oddo, P., Spada, G., and Larnicol, G.: Sea-level variability in the Mediterranean Sea from altimetry and tide gauges, Clim. Dynam., 47, 2851–2866, https://doi.org/10.1007/s00382-016-3001-2, 2016.
Bonaldo, D., Antonioli, F., Archetti, R., Bezzi, A., Correggiari, A., Davolio, S., De Falco, G., Fantini, M., Fontolan, G., Furlani, S., Gaeta, M. G., Leoni, G., Lo Presti, V., Mastronuzzi, G., Pillon, S., Ricchi, A., Stocchi, P., Samaras, A. G., Scicchitano, G., and Carniel, S.: Integrating multidisciplinary instruments for assessing coastal vulnerability to erosion and sea level rise: lessons and challenges from the Adriatic Sea, Italy, J. Coast. Conserv., 23, 19–37, https://doi.org/10.1007/s11852-018-0633-x, 2019.
Bonnefond, P., Exertier, P., Laurain, O.,Ménard, Y., Orsoni, A., Jan, G., and Jeansou, E.: Absolute calibration of Jason-1 and TOPEX/Poseidon altimeters in Corsica, Mar. Geodesy, 26, 261–284, https://doi.org/10.1080/714044521, 2003.
Bonnefond, P., Exertier, P., Laurain, O., Guinle, T., and Féménias, P.: Corsica: A 20 year multi-mission absolute altimeter calibration site, Adv. Space Res., 68, 1171–1186, https://doi.org/10.1016/j.asr.2019.09.049, 2021.
Bonnefond, P., Laurain, O., Exertier, P., Calzas, M., Guinle, T., and Picot, N.: Validating a new GNSS-based sea level instrument (CalNaGeo) at Senetosa Cape, Mar. Geodesy, 45, 121–150, https://doi.org/10.1080/01490419.2021.2013355, 2022.
Bosch, W., Dettmering, D., and Schwatke, C.: Multi-mission cross-calibration of satellite altimeters: Constructing a long-term data record for global and regional sea level change studies, Remote Sens., 6, 2255–2281, https://doi.org/10.3390/rs6032255, 2014.
Bresson, É., Arbogast, P., Aouf, L., Paradis, D., Kortcheva, A., Bogatchev, A., Galabov, V., Dimitrova, M., Morvan, G., Ohl, P., Tsenova, B., and Rabier, F.: On the improvement of wave and storm surge hindcasts by downscaled atmospheric forcing: application to historical storms, Nat. Hazards Earth Syst. Sci., 18, 997–1012, https://doi.org/10.5194/nhess-18-997-2018, 2018.
Calafat, F. M., Avgoustoglou, E., Jordà, G., Flocas, H., Zodiatis, G., Tsimplis, M. N., and Kouroutzoglou, J.: The ability of a barotropic model to simulate sea level extremes of meteorological origin in the Mediterranean Sea, including those caused by explosive cyclones, J. Geophys. Res.-Oceans, 119, 7840–7853, https://doi.org/10.1002/2014JC010360, 2014.
Cancet, M., Bijac, S., Chimot, J., Bonnefond, P., Jeansou, E., Laurain, O., Lyard, F., Bronner, E., and Féménias, P.: Regional in situ validation of satellite altimeters: calibration and cross-calibration results at the Corsican sites, Adv. Space Res., 51, 1400–1417, https://doi.org/10.1016/j.asr.2012.06.017, 2013.
Cavaleri, L., Bertotti, L., Buizza, R., Buzzi, A., Masato, V., Umgiesser, G., and Zampieri, M.: Predictability of extreme meteo-oceanographic events in the Adriatic Sea, Q. J. Roy. Meteorol. Soc., 136, 400–413, https://doi.org/10.1002/qj.567, 2010.
Cavaleri, L., Bajo, M., Barbariol, F., Bastianini, M., Benetazzo, A., Bertotti, L., Chiggiato, J., Ferrarin, C., Umgiesser, G., and Trincardi, F.: The 2019 flooding of Venice and its implications for future predictions, Oceanography, 33, 42–49, https://doi.org/10.5670/oceanog.2020.105, 2020.
Cid, A., Menéndez, M., Castanedo, S., Abascal, A. J., Méndez, F. J., and Medina, R.: Long-term changes in the frequency, intensity and duration of extreme storm surge events in southern Europe, Clim. Dynam., 46, 1503–1516, https://doi.org/10.1007/s00382-015-2659-1, 2016.
Clementi, E., Grandi, A., Pietro, P.D., Pistoia, J., Delrosso, D., Cossarini, G., Lecci, R., Drudi, M., and Fratianni, C.: Mediterranean Sea analysis and forecast (CMEMS MED-Currents, EAS5 system) [data set], London: Copernicus Monitoring Environment Marine Service (CMEMS), 2019.
Danezis, C., Nikolaidis, M., Mettas, C., Hadjimitsis, D. G., Kokosis, G., and Kleanthous, C.: Establishing an integrated permanent sea-level monitoring infrastructure towards the implementation of maritime spatial planning in Cyprus, J. Mar. Sci. Eng., 8, 861, https://doi.org/10.3390/jmse8110861, 2020.
Denamiel, C., Šepić, J., Ivanković, D., and Vilibić, I.: The Adriatic Sea and coast modelling suite: evaluation of the meteotsunami forecast component, Ocean Model., 135, 71–93, https://doi.org/10.1016/j.ocemod.2019.02.003, 2019a.
Denamiel, C., Šepić, J., Huan, X., Bolzer, C., and Vilibić, I.: Stochastic surrogate model for meteotsunami early warning system in the eastern Adriatic Sea, J. Geophys. Res.-Oceans, 124, 8485–8499, https://doi.org/10.1029/2019JC015574, 2019b.
Denamiel, C., Huan, X., and Vilibić, I.: Conceptual design of extreme sea-level early warning systems based on uncertainty quantification and engineering optimization methods, Front. Mar. Sci., 8, 650279, https://doi.org/10.3389/fmars.2021.650279, 2021.
Dieng, H. B., Cazenave, A., Gouzenes, Y., and Sowd, B. A.: Trends and inter-annual variability of altimetry-based coastal sea level in the Mediterranean Sea: Comparison with tide gauges and models, Adv. Space Res., 68, 3279–3290, https://doi.org/10.1016/j.asr.2021.06.022, 2021.
Dogan, G. G., Annunziato, A., Papadopoulos, G. A., Guler, H. G., Yalciner, A. C., Cakir, T. E., Sozdinler, C. O., Ulutas, E., Arikawa, T., Suzen, M. L., Guler, I., Probst, P., Kânoğlu, U., and Synolakis, C.: The 20th July 2017 Bodrum–Kos Tsunami field survey, Pure Appl. Geophys., 176, 2925–2949, https://doi.org/10.1007/s00024-019-02151-1, 2019.
Dogan, G. G., Yalciner, A. C., Yuksel, Y., Ulutaş, E., Polat, O., Güler, I., Şahin, C., Tarih, A., and Kânoğlu, U.: Correction to: The 30 October 2020 Aegean Sea Tsunami: Post-event field survey along Turkish coast, Pure Appl. Geophys., 178, 2393, https://doi.org/10.1007/s00024-021-02753-8, 2021.
Drago, A.: Sea level variability and the “Milghuba” seiche oscillations in the northern coast of Malta, Central Mediterranean, Phys. Chem. Earth, 34, 948–970, https://doi.org/10.1016/j.pce.2009.10.0022009, 2009.
Drago, A.: Sea level measurements in Malta, in: IOC Workshop Report no. 176 “MedGLOSS Workshop and Coordination Meeting for the Pilot Monitoring Network System of Systematic Sea Level Measurements in the Mediterranean and Black Seas”, IOLR, Haifa, Israel 15–17 May 2000, Annex IVb, 8–11, 2000.
El-Fishawi, N. M.: Coastal erosion in relation to sea level changes, subsidence and river discharge, Nile Delta coast, Acta Mineral Petrogr., 30, 161–171, 1989.
El-Geziry, T. M. and Said, M. A.: Spatial variations of sea level along the Egyptian Mediterranean coast, Athens J. Medit. Studies, 6, 141–154, 2020.
Escudier, R., Clementi, E., Cipollone, A., Pistoia, J., Drudi, M., Grandi, A., Lyubartsev, V., Lecci, R., Aydogdu, A., Delrosso, D., Omar, M., Masina, S., Coppini, G., and Pinardi, N.: A High Resolution Reanalysis for the Mediterranean Sea, Front. Earth Sci., 9, https://doi.org/10.3389/feart.2021.702285, 2021.
Esselborn, S., Rudenko, S., and Schöne, T.: Orbit-related sea level errors for TOPEX altimetry at seasonal to decadal timescales, Ocean Sci., 14, 205–223, https://doi.org/10.5194/os-14-205-2018, 2018.
Ferrarin, C., Valentini, A., Vodopivec, M., Klaric, D., Massaro, G., Bajo, M., De Pascalis, F., Fadini, A., Ghezzo, M., Menegon, S., Bressan, L., Unguendoli, S., Fettich, A., Jerman, J., Ličer, M., Fustar, L., Papa, A., and Carraro, E.: Integrated sea storm management strategy: the 29 October 2018 event in the Adriatic Sea, Nat. Hazards Earth Syst. Sci., 20, 73–93, https://doi.org/10.5194/nhess-20-73-2020, 2020.
Ferrarin, C., Bajo, M., Benetazzo, A., Cavaleri, L., Chiggiato, J., Davison, S., Davolio, S., Lionello, P., Orlić, M., and Umgiesser, G.: Local and large-scale controls of the exceptional Venice floods of November 2019, Prog. Oceanogr., 197, 102628, https://doi.org/10.1016/j.pocean.2021.102628, 2021.
Flanders Marine Institute (VLIZ): Intergovernmental Oceanographic Commission (IOC) Sea level station monitoring facility, http://www.ioc-sealevelmonitoring.org (last access: 14 December 2021), 2021.
Fokaefs, A. and Papadopoulos, G.: Tsunami hazard in the eastern Mediterranean: strong earthquakes in Cyprus and the Levantine Sea, Nat. Hazards, 40, 503–526, https://doi.org/10.1007/s11069-006-9011-3, 2007.
Frappart, F., Roussel, N., Biancale, R., Benjamin, J. J. M., Mercier, F., Perosanz, F., Pasquin, J. G., Davila, J. M., Gomez, B. P., Gomez, C. G., Bravo, R. L., Gomez, A. T., Ripoll, J. G., Pajares, M. H., Lino, M. S., Bonnefond, P., and Casanova, I. V.: The 2013 Ibiza calibration campaign of Jason-2 and SARAL altimeters, Mar. Geodesy, 38, 219–232, https://doi.org/10.1080/01490419.2015.1008711, 2015.
Fu, L. L. and Haines, B. J.: The challenges in long-term altimetry calibration for addressing the problem of global sea level change, Adv. Space Res., 51, 1284–1300, https://doi.org/10.1016/j.asr.2012.06.005, 2013.
García-Valdecasas, J., Pérez Gómez, B., Molina, R., Rodríguez, A., Rodríguez, D., Pérez, S., Campos, A., Rodríguez Rubio, P., Gracia, S., Ripollés, L., Terrés Nicoli, J. M., de los Santos, F. J., and Álvarez Fanjul, E.: Operational tool for characterizing high-frequency sea level oscillations, Nat. Hazards, 106, 1149–1167, https://doi.org/10.1007/s11069-020-04316-x, 2021.
Gomis, D., Tsimplis, M., Marcos, M., Fenoglio-Marc, L., Pérez Gómez, B., Raicich, F., Vilibic, I., Wöppelmann, G., and Monserrat, S.: Mediterranean sea-level variability and trends, in: Climate of the Mediterranean region: From the Past to the Future, edited by: Lionello, P., Elsevier Insights, 257–299, https://doi.org/10.1016/B978-0-12-416042-2.00004-5, 2012.
Gouzenes, Y., Léger, F., Cazenave, A., Birol, F., Bonnefond, P., Passaro, M., Nino, F., Almar, R., Laurain, O., Schwatke, C., Legeais, J.-F., and Benveniste, J.: Coastal sea level rise at Senetosa (Corsica) during the Jason altimetry missions, Ocean Sci., 16, 1165–1182, https://doi.org/10.5194/os-16-1165-2020, 2020.
Grases, A., Gracia, V., García-León, M., Lin-Ye, J., and Sierra, J. P.: Coastal flooding and erosion under a changing climate: Implications at a low-lying coast (Ebro Delta), Water, 12, 346, https://doi.org/10.3390/w12020346, 2020.
Gusiakov, V. K.: Meteotsunamis at global scale: problem of event identification, parametrization and cataloguing, Nat. Hazards, 106, 1105–1123, https://doi.org/10.1007/s11069-020-04230-2, 2021.
Hamden, M. H. and Md Din, A. H.: A review of advancement of hydrographic surveying towards ellipsoidal referenced surveying technique, IOP Conf. Ser. Earth Environ. Sci., 169, 012019, https://doi.org/10.1088/1755-1315/169/1/012019, 2018.
Hereher, M. E.: Coastal vulnerability assessment for Egypt's Mediterranean coast, Geomatics, Nat. Hazards Risk, 6, 342–355, https://doi.org/10.1080/19475705.2013.845115, 2015.
Holgate, S., Foden, P., Pugh, J., and Woodworth, P.: Real time sea-level data transmission from tide gauges for tsunami monitoring and long-term sea level rise observations, J. Oper. Oceanogr, 1, 3–8, https://doi.org/10.1080/1755876X.2008.11081883, 2008.
Holgate, S. J., Matthews, A., Woodworth, P. L., Rickards, L. J., Tamisiea, M. E., Bradshaw, E., Foden, P. R., Gordon, K. M., Jevrejeva, S., and Pugh, J.: New data systems and products at the Permanent Service for Mean Sea Level, J. Coastal Res., 29, 493–504, https://doi.org/10.2112/JCOASTRES-D-12-00175.1, 2013.
Jabnoun, R. and Harzallah, A.: An estimate of the sea level trend along the Tunisia coast, Bull. Inst. Natn. Scien. Tech. Mer de Salammbô̂, Vol. 47, 165–171, 2020.
Jansà, A. and Ramis, C.: The Balearic rissaga: from pioneering research to present-day knowledge, Nat. Hazards, 106, 1269–1297, https://doi.org/10.1007/s11069-020-04221-3, 2021.
Jansà, A., Monserrat, S., and Gomis, D.: The rissaga of 15 June 2006 in Ciutadella (Menorca), a meteorological tsunami, Adv. Geosci., 12, 1–4, https://doi.org/10.5194/adgeo-12-1-2007, 2007.
Le Traon, P. Y.: From satellite altimetry to Argo and operational oceanography: three revolutions in oceanography, Ocean Sci., 9, 901–915, https://doi.org/10.5194/os-9-901-2013, 2013.
Le Traon, P. Y., Reppucci, A., Alvarez Fanjul, E., Aouf, L., Behrens, A., Belmonte, M., Bentamy, A., Bertino, L., Brando, V. E., Kreiner, M. B., Benkiran, M., Carval, T., Ciliberti, S. A., Claustre, H., Clementi, E., Coppini, G., Cossarini, G., De Alfonso Alonso Muñoyerro, M., Delamarche, A., Dibarboure, G., Dinessen, F., Drevillon, M., Drillet, Y., Faugere, Y., Fernández, V., Fleming, A., Garcia-Hermosa, M. I., Sotillo, M. G., Garric, G., Gasparin, F., Giordan, C., Gehlen, M., Gregoire, M. L., Guinehut, S., Hamon, M., Harris, C., Hernandez, F., Hinkler, J. B., Hoyer, J., Karvonen, J., Kay, S., King, R., Lavergne, T., Lemieux-Dudon, B., Lima, L., Mao, C., Martin, M. J., Masina, S., Melet, A., Buongiorno Nardelli, B., Nolan, G., Pascual, A., Pistoia, J., Palazov, A., Piolle, J. F., Pujol, M. I., Pequignet, A. C., Peneva, E., Pérez Gómez, B., Petit de la Villeon, L., Pinardi, N., Pisano, A., Pouliquen, S., Reid, R., Remy, E., Santoleri, R., Siddorn, J., She, J., Staneva, J., Stoffelen, A., Tonani, M., Vandenbulcke, L., von Schuckmann, K., Volpe, G., Wettre, C., and Zacharioudaki, A.: From observation to information and users: The Copernicus Marine Service perspective, Front. Mar. Sci., 6, 234, https://doi.org/10.3389/fmars.2019.00234, 2019.
Lemon, D. D., Chave, R. A. J., Clarke, M. R., Curran, T. A., Hinds, A., Thorn, A., Thomson, A., and Badger, C. J.: Real-time monitoring of currents and water level at second narrows to improve port efficiency in Vancouver harbour, Oceans 2003, Celebrating the Past, Teaming Toward the Future (IEEE Cat. No. 03CH37492), 231–237, https://doi.org/10.1109/OCEANS.2003.178559, 2003.
Letetrel, C., Marcos, M., Miguez, B. M., and Wöppelmann, G.: Sea level extremes in Marseille (NW Mediterranean) during 1885–2008, Cont. Shelf Res., 30, 1267–1274, https://doi.org/10.1016/j.csr.2010.04.003, 2010.
Ličer, M., Mourre, B., Troupin, C., Krietemeyer, A., Jansá, A., and Tintoré, J.: Numerical study of Balearic meteotsunami generation and propagation under synthetic gravity wave forcing, Ocean Model., 111, 38–45, https://doi.org/10.1016/j.ocemod.2017.02.001, 2017.
Lionello, P., Conte, D., Marzo, L., and Scarascia, L.: The contrasting effect of increasing mean sea level and decreasing storminess on the maximum water level during storms along the coast of the Mediterranean Sea in the mid 21st century, Global Planet. Change, 151, 80–91, https://doi.org/10.1016/j.gloplacha.2016.06.012, 2017.
Lionello, P., Barriopedro, D., Ferrarin, C., Nicholls, R. J., Orlić, M., Raicich, F., Reale, M., Umgiesser, G., Vousdoukas, M., and Zanchettin, D.: Extreme floods of Venice: characteristics, dynamics, past and future evolution (review article), Nat. Hazards Earth Syst. Sci., 21, 2705–2731, https://doi.org/10.5194/nhess-21-2705-2021, 2021.
Loizides, G., Papazachariou, D., and Zodiatis, G.: Data analysis of the CYCOFOS Paphos Sea Level Station-MedGloss Network 2001–2010. internal report, Oceanography Center, University of Cyprus, 23 August 2010.
Makris, C., Androulidakis, Y., Karambas, T., Papadimitriou, A., Metallinos, A., Kontos, Y., Baltikas, V., Chondros, M., Krestenitis, Y., Tsoukala, V., and Memos, C.: Integrated modelling of sea-state forecasts for safe navigation and operational management in ports: Application in the Mediterranean Sea, Appl. Math. Model., 89, 1206–1234, https://doi.org/10.1016/j.apm.2020.08.015, 2021.
Maramai, A., Brizuela, B., and Graziani, L.: The Euro-Mediterranean Tsunami Catalogue, Ann. Geophys., 57, 4, https://doi.org/10.4401/ag-6437, 2014.
Maramai, A., Graziani L., and Brizuela B.: Euro-Mediterranean Tsunami Catalogue (EMTC), version 2.0, Istituto Nazionale di Geofisica e Vulcanologia (INGV), https://doi.org/10.13127/tsunami/emtc.2.0, 2019.
Marcos, M., Monserrat, S., Medina, R., Orfila, A., and Olabarrieta, M.: External forcing of meteorological tsunamis at the coast of the Balearic Islands, Phys. Chem. Earth, 34, 838–947, https://doi.org/10.1016/j.pce.2009.10.001, 2009a.
Marcos, M., Tsimplis, M. N., and Shaw, A. G. P.: Sea level extremes in southern Europe, J. Geophys. Res., 114, C01007, https://doi.org/10.1029/2008JC004912, 2009b.
Marcos, M., Jordà, G., Gomis, D., and Pérez Gómez, B.: Changes in storm surges in southern Europe from a regional model under climate change scenarios, Global Planet. Change, 77, 116–128, https://doi.org/10.1016/j.gloplacha.2011.04.002, 2011.
Marcos, M., Calafat, F. M., Berihuete, A., and Dangendorf, S.: Long-term variations in global sea level extremes, J. Geophys. Res.-Oceans, 120, 8115–8134, https://doi.org/10.1002/2015JC011173, 2015.
Marcos, M. and Woodworth, P. L.: Spatiotemporal changes in extreme sea levels along the coasts of the North Atlantic and the Gulf of Mexico, J. Geophys. Res.-Oceans, 122, 7031–7048, https://doi.org/10.1002/2017JC013065, 2017.
Marcos, M., Puyol, B., Amores, A., Pérez Gómez, B., Fraile, M. A., and Talke, A.: Historical tide gauge sea-level observations in Alicante and Santander (Spain) since the 19th century, Geosci. Data J., 8, 144–153, https://doi.org/10.1002/gdj3.112, 2021.
Marrobbio Project Report: Creation of a prediction station for Marrobbio events to be installed in the fishing port of Mazara del Vallo, funded by SFOP 2004 – Mis. 4.17b (Cod. 1999.IT.16.1.PO.011/4.17b/8.3.7/0082), 2007.
Martinez-Benjamin, J. J., Martinez-Garcia, M., Lopez, S. G., Andres, A. N., Pozuelo, F. B., Infantes, M. E., Lopez-Marco, J., Davila, J. M., Pasquin, J. G., Silva, C. G., Bonnefond, P., Laurain, O., Isanta, A. M. B., Castellon, M. A. O., Lopez, J. T., Pérez Gomez, B., Álvarez Fanjul, E., Velasco, G. R., Gomis, D., Marcos, M., Menard, Y., Jan, G., Jeansou, E., Lyard, F., and Roblou, L.: Ibiza absolute calibration experiment: Survey and preliminary results, Mar. Geodesy, 27, 657–681, https://doi.org/10.1080/01490410490883342, 2004.
Masina, M. and Lamberti, A.: A nonstationary analysis for the Northern Adriatic extreme sea levels, J. Geophys. Res.-Oceans, 118, 3999–4016, https://doi.org/10.1002/jgrc.20313, 2013.
Mel, R. and Lionello, P.: Verification of an ensemble prediction system for storm surge forecast in the Adriatic Sea, Ocean Dynam., 64, 1803–1814, https://doi.org/10.1007/s10236-014-0782-x, 2014.
Međugorac, I., Pasarić, M., and Güttler, I.: Will the wind associated with the Adriatic storm surges change in future climate?, Theor. Appl. Climatol., 143, 1–18, https://doi.org/10.1007/s00704-020-03379-x, 2021.
Medvedev, I. P., Vilibić, I., and Rabinovich, A. B.: Tidal resonance in the Adriatic Sea: Observational evidence, J. Geophys. Res.-Oceans, 125, e2020JC016168, https://doi.org/10.1029/2020JC016168, 2020.
Monserrat, S., Ramis, C., and Thorpe, A. J.: Large-amplitude pressure oscillations in the Western Mediterranean, Geophys. Res. Lett., 18, 183–186, https://doi.org/10.1029/91GL00234, 1991.
Monserrat, S., Rabinovich, A. B., and Casas, B.: On the reconstruction of the transfer function for atmospherically generated seiches, Geophys. Res. Lett., 25, 2197–2200, https://doi.org/10.1029/98GL01506, 1998.
Monserrat, S., Vilibić, I., and Rabinovich, A. B.: Meteotsunamis: atmospherically induced destructive ocean waves in the tsunami frequency band, Nat. Hazards Earth Syst. Sci., 6, 1035–1051, https://doi.org/10.5194/nhess-6-1035-2006, 2006.
Mourre, B., Santana, A., Buils, A., Gautreau, L., Ličer, M., Jansà, A., Casas, B., Amengual, B., and Tintoré, J.: On the potential of ensemble forecasting for the prediction of meteotsunamis in the Balearic Islands: sensitivity to atmospheric model parameterizations, Nat. Hazards, 106, 1315–1336, https://doi.org/10.1007/s11069-020-03908-x, 2021.
Novellino, A., D'Angelo, P., Benedetti, G., Manzella, G., Gorringe, P., Schaap, D., Pouliquen, S., and Rickards, L.: European Marine Observation Data Network – EMODnet Physics. OCEANS 2015 – Genova, 1–6, https://doi.org/10.1109/OCEANS-Genova.2015.7271548, 2015.
Oaie, G., Seghedi, A., and Radulescu, V.: Natural marine hazards in the Black Sea and the system of their monitoring and real-time warning, Geo-Eco-Marina, 22, 5–28, https://doi.org/10.5281/zenodo.889593, 2016.
Okal, E. A., Synolakis, C. E., Uslu, B., Kalligeris, N., and Voukouvalas, E.: The 1956 earthquake and tsunami in Amorgos, Greece, Geophys. J. Int., 178, 1533–1554, https://doi.org/10.1111/j.1365-246X.2009.04237.x, 2009.
Okal, E. A.: On the possibility of seismic recording of meteotsunamis, Nat. Hazards, 106, 1125–1147, https://doi.org/10.1007/s11069-020-04146-x, 2021.
Oppenheimer, M., Glavovic, B. C., Hinkel, J., van de Wal, R., Magnan, A. K., Abd-Elgawad, A., Cai, R., Cifuentes-Jara, M., DeConto, R. M., Ghosh, T., Hay, J., Isla, F., Marzeion, B., Meyssignac, B., and Sebesvari, Z.: Sea Level Rise and Implications for Low-Lying Islands, Coasts and Communities, in: IPCC Special Report on the Ocean and Cryosphere in a Changing Climate, edited by: Pörtner, H.-O., Roberts, D. C., Masson-Delmotte, V., Zhai, P., Tignor, M., Poloczanska, E., Mintenbeck, K., Alegría, A., Nicolai, M., Okem, A., Petzold, J., Rama, B., and Weyer, N. M., Cambridge University Press, Cambridge, UK and New York, NY, USA, 321–445, https://doi.org/10.1017/9781009157964.006, 2019.
Orfila, A., Balle, S., and Simarro, G.: Topographic enhancement of long waves generated by an idealized moving pressure system, Science, 75, 595–693, https://doi.org/10.3989/scimar.2011.75n3595, 2011.
Orlić, M.: The first attempt at cataloguing tsunami-like waves of meteorological origin in Croatian coastal waters, Acta Adriat., 56, 83–96, 2015.
Orlić, M. and Pasarić, M: Recent data on high-frequency sea-level variability in Vela Luka and Stari Grad Bays, International Symposium on Meteotsunamis, 30th Anniversary of the Great Flood of Vela Luka (21 June 1978), Book of Abstracts, Vela Luka, Croatia, p. 36, 2008.
Orlić, M. and Šepić, J.: Meteorological tsunamis in the Adriatic Sea – catalogue of meteorological tsunamis in Croatian coastal waters, https://jadran.izor.hr/~sepic/meteotsunami_catalogue/ (last access: 21 September 2021), 2019.
Orlić, M., Belušić, D., Janeković, I., and Pasarić, M.: Fresh evidence relating the great Adriatic surge of 21 June 1978 to mesoscale atmospheric forcing, J. Geophys. Res., 115, C06011, https://doi.org/10.1029/2009JC005777, 2010.
Pascual, J. and Salat, J.: Monthly sea level anomaly based on 2 h data from L'Estartit meteorological station II (NW Mediterranean) since 1990, PANGAEA, https://doi.org/10.1594/PANGAEA.902604, 2019.
Papadopoulos, G. and Papageorgiou, A.: Large earthquakes and tsunamis in the Mediterranean region and its connected seas, in: Extreme Natural Hazards, Disaster Risks and Societal Implications, Cambridge University Press, Cambridge, 252–266, https://doi.org/10.1017/CBO9781139523905.025, 2012.
Papadopoulos, G., Gràcia, E., Urgeles, R., Sallares, V., De Martini, P. M., Pantosti, D., González, M., Yalciner, A., Mascle, J., Sakellariou, D., Salamon, A., Tinti, S., Fokaefs, A., Camerlenghi, A., Novikova, T., and Papageorgiou, A.: Historic and pre-historic tsunamis in the Mediterranean and its connected seas: a review on documentation, geological signatures, generation mechanisms and coastal impacts, Mar. Geol., 354, 81–109, https://doi.org/10.1016/j.margeo.2014.04.014, 2014.
Papazachariou, D.: Estimation of the lowest astronomical tide and the lowest low tide at tide-gauge stations in Cyprus. Internal Report, Oceanography Center, University of Cyprus, 10 October, 2014.
Papazachariou, D., Zodiatis, G., Nikolaidis, A., Stylianou, S., and Arabelos, D.: Satellite altimetry and tide gauge data for monitoring sea level changes in the Eastern Mediterranean, Geophys. Res. Abstracts, 16, EGU2014-9582, 2014.
Pasarić, M., Pasarić, Z., and Orlić, M.: Response of the Adriatic Sea level to the air pressure and wind forcing at low frequencies (0.01–0.1 cpd), J. Geophys. Res., 105, 11423–11439, https://doi.org/10.1029/2000JC900023, 2000.
Pashova, L.: Assessment of the sea level change on different timescales from Varna and Burgas tide gauge data, C. R. Acad. Bulg. Sci., 65, 193–202, 2012.
Peng, D., Feng, L., Larson, K. M., and Hill, E. M.: Measuring coastal absolute sea-level changes using GNSS interferometric reflectometry, Remote Sens., 13, 4319, https://doi.org/10.3390/rs13214319, 2021.
Pérez, B., Brouwer, R., Beckers, J., Paradis, D., Balseiro, C., Lyons, K., Cure, M., Sotillo, M. G., Hackett, B., Verlaan, M., and Fanjul, E. A.: ENSURF: multi-model sea level forecast – implementation and validation results for the IBIROOS and Western Mediterranean regions, Ocean Sci., 8, 211–226, https://doi.org/10.5194/os-8-211-2012, 2012.
Pérez, B., Payo, A., López, D., Woodworth, P. L., and Alvarez Fanjul, E.: Overlapping sea level time series measured using different technologies: an example from the REDMAR Spanish network, Nat. Hazards Earth Syst. Sci., 14, 589–610, https://doi.org/10.5194/nhess-14-589-2014, 2014.
Pérez Gómez, B., Alvarez Fanjul, E., Pérez Rubio, S., de Alfonso, M., and Vela, J.: Use of tide gauge data in operational oceanography and sea level hazard warning systems, J. Oper. Oceanogr., 6, 1–18, https://doi.org/10.1080/1755876X.2013.11020147, 2013.
Pérez Gómez, B., García-León M., García-Valdecasas, J., Clementi, E., Mösso Aranda, C., Pérez-Rubio, S., Masina, S., Coppini, G., Molina-Sánchez, R., Muñoz-Cubillo, A., García Fletcher, A., Sánchez González, J. F., Sánchez-Arcilla, A., and Álvarez-Fanjul, E.: Understanding sea level processes during Western Mediterranean storm Gloria, Front. Mar. Sci., 8, 647437, https://doi.org/10.3389/fmars.2021.647437, 2021.
Pérez González, I., Pérez Gómez, B., Sotillo, M. G., and Álvarez Fanjul, E.: Towards a new sea level forecast system in Puertos del Estado. Extended proceedings, in: Proceedings of the 8th EuroGOOS Conference, ISBN 978-2-9601883-3-2, Bergen, 2017.
Pasarić, M. and Orlić, M.: Long-term meteorological preconditioning of the North Adriatic coastal floods, Cont. Shelf Res., 21, 263–278, https://doi.org/10.1016/S0278-4343(00)00078-9, 2001.
Proudman, J.: The effects on the sea of changes in atmospheric pressure, Geophys. Suppl. Mon. Not. R. Astron. Soc., 2, 197–209, https://doi.org/10.1111/j.1365-246X.1929.tb05408.x, 1929.
Pugh, D. and Woodworth, P.: Sea-Level Science: Understanding Tides, Surges, Tsunamis and Mean Sea-Level Changes, Cambridge University Press, Cambridge, https://doi.org/10.1017/CBO9781139235778, 2014.
Quartly, G. D., Chen, G., Nencioli, F., Morrow, R., and Picot, N.: An overview of requirements, procedures and current advances in the calibration/validation of radar altimeters, Remote Sens., 13, 125, https://doi.org/10.3390/rs13010125, 2021.
Rabinovich, A. B.: Twenty-seven years of progress in the science of meteorological tsunamis following the 1992 Daytona Beach event, Pure Appl. Geophys., 177, 1193–1230, https://doi.org/10.1007/s00024-019-02349-3, 2020.
Rabinovich, A. B. and Monserrat, S.: Meteorological tsunamis near the Balearic and Kuril Islands: descriptive and statistical analysis, Nat. Hazards, 13, 55–90, https://doi.org/10.1007/BF00156506, 1996.
Raicich, F.: Recent evolution of sea-level extremes at Trieste (Northern Adriatic), Cont. Shelf Res., 23, 225–235, htpps://doi.org/10.1016/S0278-4343(02)00224-8, 2003.
Raicich, F.: Sea level observations at Trieste Molo Sartorio (Italy), SEANOE, https://doi.org/10.17882/62758, 2019.
Renault, L., Vizoso, G., Jansà, A., Wilkin, J., and Tintoré, J.: Toward the predictability of meteotsunamis in the Balearic Sea using regional nested atmosphere and ocean models, Geophys. Res. Lett., 38, L10601, https://doi.org/10.1029/2011GL047361, 2011.
Reimann, L., Vafeidis, A. T., Brown, S., Hinkel, J., and Tol, R. S. J.: Mediterranean UNESCO World Heritage at risk from coastal flooding and erosion due to sea-level rise, Nat. Comm., 9, 4161, https://doi.org/10.1038/s41467-018-06645-9, 2018.
Romero, R., Vich, M., and Ramis, C.: A pragmatic approach for the numerical prediction of meteotsunamis in Ciutadella harbour (Balearic Islands), Ocean Model., 142, 101441, https://doi.org/10.1016/j.ocemod.2019.101441, 2019.
Rosen, D. S. and Aarup, T.: IOC/CIESM MedGLOSS Pilot Network Workshop and Coordination Meeting, Haifa, 15–17 May 2000, IOC/UNESCO Report 176, 120 pp., 2002.
Samaras, A. G., Karambas, T. V., and Archetti, R.: Simulation of tsunami generation, propagation and coastal inundation in the Eastern Mediterranean, Ocean Sci., 11, 643–655, https://doi.org/10.5194/os-11-643-2015, 2015.
Schindelé, F., Loevenbruck, A., and Hébert, H.: Strategy to design the sea-level monitoring networks for small tsunamigenic oceanic basins: the Western Mediterranean case, Nat. Hazards Earth Syst. Sci., 8, 1019–1027, https://doi.org/10.5194/nhess-8-1019-2008, 2008.
Schindellé, F., Gailler, A., Hébert, H., Loevenbruck, A., Gutierrez, E., Monnier, A., Roudil, P., Reimond, D. and Rivera, L.: Implementation and challenges of the tsunami warning system in the Western Mediterranean, Pure Appl. Geophys., 172, 821–833, https://doi.org/10.1007/s00024-014-0950-4, 2015.
Salat, J., Pascual, J., Flexas, M. M., Chin, T. M., and Vázquez-Cuervo, J.: Forty-five years of oceanographic and meteorological observations at a coastal station in the NW Mediterranean: a ground truth for satellite observations, Ocean Dynam, 69, 1067–1084, https://doi.org/10.1007/s10236-019-01285-z, 2019.
Šepić, J. and Vilibić, I.: The development and implementation of a real-time meteotsunami warning network for the Adriatic Sea, Nat. Hazards Earth Syst. Sci., 11, 83–91, https://doi.org/10.5194/nhess-11-83-2011, 2011.
Šepić, J., Vilibić, I., and Monserrat, S.: Teleconnections between the Adriatic and the Balearic meteotsunamis, Phys. Chem. Earth, 34, 928–937, https://doi.org/10.1016/j.pce.2009.08.007, 2009.
Šepić, J., Vilibić, I., Jordà, G., and Marcos, M.: Mediterranean Sea level forced by atmospheric pressure and wind: Variability of the present climate and future projections for several period bands, Global Planet. Change, 86, 20–30, https://doi.org/10.1016/j.gloplacha.2012.01.008, 2012.
Šepić, J., Međugorac, I., Janeković, I., Dunić, N., and Vilibić, I.: Multi-meteotsunami event in the Adriatic Sea generated by atmospheric disturbances of 25–26 June 2014, Pure Appl. Geophys., 173, 4117–4138, https://doi.org/10.1007/s00024-016-1249-4, 2016a.
Šepić, J., Vilibić, I., and Monserrat, S.: Quantifying the probability of meteotsunami occurrence from synoptic atmospheric patterns, Geophys. Res. Lett., 43, 10377–10384, https://doi.org/10.1002/2016GL070754, 2016b.
Šepić, J., Vilibić, I., Rabinovich, A. B., and Tinti, S.: Meteotsunami (“Marrobbio”) of 25–26 June 2014 on the southwestern coast of Sicily, Italy, Pure Appl. Geophys., 175, 1573–1593, https://doi.org/10.1007/s00024-018-1827-8, 2018.
Spada, G. and Galassi, G.: New estimates of secular sea level rise from tide gauge data and GIA modelling, Geophys. J. Int., 191, 1067–1094, https://doi.org/10.1111/j.1365-246X.2012.05663.x, 2012.
Sotillo, M. G., Cailleau, S., Lorente, P., Levier, B., Aznar, R., Reffray, G., Amo-Baladrón, A., Chanut, J., Benkiran, M., and Alvarez-Fanjul, E.: The MyOcean IBI ocean forecast and reanalysis systems: operational products and roadmap to the future Copernicus Service, J. Operat. Oceanogr., 8, 1–18, https://doi.org/10.1080/1755876X.2015.1014663, 2015.
Sørensen, M. B., Spada, M., Babeyko, A., Wiemer, S., and Grünthal, G.: Probabilistic tsunami hazard in the Mediterranean Sea, J. Geophys. Res., 117, B01305, https://doi.org/10.1029/2010JB008169, 2012.
Taibi, H. and Haddad, M.: Estimating trends of the Mediterranean Sea level changes from tide gauge and satellite altimetry data (1993–2015), J. Oceanol. Limnol., 37, 1176–1185, https://doi.org/10.1007/s00343-019-8164-3, 2019.
Tinti, S. and Maramai, A.: Catalogue of tsunamis generated in Italy and in Cote d'Azur, France: a step towards a unified catalogue of tsunamis in Europe, Ann. Geophys., 39, 1253–1299, 1996.
Tinti, S., Maramai, A., and Graziani, L.: A new version of the European tsunami catalogue: updating and revision, Nat. Hazards Earth Syst. Sci., 1, 255–262, https://doi.org/10.5194/nhess-1-255-2001, 2001.
Tinti, S., Manucci, A., Pagnoni, G., Armigliato, A., and Zaniboni, F.: The 30 December 2002 landslide-induced tsunamis in Stromboli: sequence of the events reconstructed from the eyewitness accounts, Nat. Hazards Earth Syst. Sci., 5, 763–775, https://doi.org/10.5194/nhess-5-763-2005, 2005.
Tintoré, J., Vizoso, G., Casas, B., Heslop, E., Pascual, A., Orfila, A., Ruiz, S., Martínez-Ledesma, M., Torner, M., Cusí, S., Diedrich, A., Balaguer, P., Gómez-Pujol, L., Álvarez-Ellacuria, A., Gómara, S., Sebastian, K., Lora, S., Renault, L., Juzà, M., Álvarez, D., March, D., Garau, B., Cañellas, T., Roque, D., Lizarán, I., Pitarch, S., Carrasco, M. A., Lana, A., Mason, E., Escudier, R., Conti, D. S., Barceló, B., Alemany, F., Reglero, P., Massuti, E., Vélez-Belchí, P., Ruiz, J., Oguz, T., Gómez, M., Álvarez Fanjul, E., Ansorena, L., and Manriquez, M.: The Balearic Islands Coastal Ocean Observing and Forecasting System Responding to Science, Technology and Society Needs, Mar. Technol. Soc. J., 47, 101–117, https://doi.org/10.4031/MTSJ.47.1.10, 2013.
Tintoré, J., Pinardi, N., Álvarez-Fanjul, E., Aguiar, E., Álvarez-Berastegui, D., Bajo, M., Balbin, R., Bozzano, R., Nardelli, B. B., Cardin, V., Casas, B., Charcos-Llorens, M., Chiggiato, J., Clementi, E., Coppini, G., Coppola, L., Cossarini, G., Deidun, A., Deudero, S., D'Ortenzio, F., Drago, A., Drudi, M., El Serafy, G., Escudier, R., Farcy, P., Federico, I., Fernández, J. G., Ferrarin, C., Fossi, C., Frangoulis, C., Galgani, F., Gana, S., García Lafuente, J., Sotillo, M. G., Garreau, P., Gertman, I., Gómez-Pujol, L., Grandi, A., Hayes, D., Hernández-Lasheras, J., Herut, B., Heslop, E., Hilmi, K., Juza, M., Kallos, G., Korres, G., Lecci, R., Lazzari, P., Lorente, P., Liubartseva, S., Louanchi, F., Malacic, V., Mannarini, G., March, D., Marullo, S., Mauri, E., Meszaros, L., Mourre, B., Mortier, L., Muñoz-Mas, C., Novellino, A., Obaton, D., Orfila, A., Pascual, A., Pensieri, S., Pérez Gómez, B., Pérez Rubio, S., Perivoliotis, L., Petihakis, G., de la Villéon, L. P., Pistoia, J., Poulain, P. M., Pouliquen, S., Prieto, L., Raimbault, P., Reglero, P., Reyes, E., Rotllan, P., Ruiz, S., Ruiz, J., Ruiz, I., Ruiz-Orejón, L. F., Salihoglu, B., Salon, S., Sammartino, S., Sánchez Arcilla, A., Sánchez-Román, A., Sannino, G., Santoleri, R., Sardá R., Schroeder, K., Simoncelli, S., Sofianos, S., Sylaios, G., Tanhua, T., Teruzzi, A., Testor, P., Tezcan, D., Torner, M., Trotta, F., Umgiesser, G., von Schuckmann, K., Verri, G., Vilibic, I., Yucel, M., Zavatarelli, M., and Zodiatis, G.: Challenges for Sustained Observing and Forecasting Systems in the Mediterranean Sea, Front. Mar. Sci., 6, 568, https://doi.org/10.3389/fmars.2019.00568, 2019.
Tsimplis, M. N.: Tidal oscillations in the Aegean and the Ionian Seas, Estuar. Coast. Shelf Sci., 39, 201–208, https://doi.org/10.1006/ecss.1994.1058, 1994.
Tsimplis, M. N., Proctor, R., and Flather, R. A.: A 2-dimensional tidal model for the Mediterranean Sea, J. Geophys. Res., 100, 16223–16239, https://doi.org/10.1029/95JC01671, 1995.
Tsimplis, M. N. and Spencer, N. E.: Collection and analysis of monthly mean sea level data in the Mediterranean and the Black Sea, J. Coastal Res., 13, 534–544, 1997.
Tsimplis, M. N., Álvarez-Fanjul, E., Gomis, D., Fenoglio-Marc, L., and Pérez Gómez, B.: Mediterranean sea level trends: atmospheric pressure and wind contribution, Geophys. Res. Lett., 32, L20602, https://doi.org/10.1029/2005GL023867, 2005.
Umgiesser, G., Bajo, M., Ferrarin, C., Cucco, A., Lionello, P., Zanchettin, D., Papa, A., Tosoni, A., Ferla, M., Coraci, E., Morucci, S., Crosato, F., Bonometto, A., Valentini, A., Orlić, M., Haigh, I. D., Nielsen, J. W., Bertin, X., Fortunato, A. B., Pérez Gómez, B., Alvarez Fanjul, E., Paradis, D., Jourdan, D., Pasquet, A., Mourre, B., Tintoré, J., and Nicholls, R. J.: The prediction of floods in Venice: methods, models and uncertainty (review article), Nat. Hazards Earth Syst. Sci., 21, 2679–2704, https://doi.org/10.5194/nhess-21-2679-2021, 2021.
UNESCO/IOC: North-East Atlantic, the Mediterranean and Connected Seas Tsunami Warning and Mitigation System, NEAMTWS, Implementation Plan (Third Session of the Intergovernmental Coordination Group for the North-East Atlantic, the Mediterranean and Connected Seas Tsunami Warning and Mitigation System, NEAMTWS), IOC Technical Series No. 73, 2007.
UNESCO/IOC: Interim operational users guide for the tsunami early warning and mitigation system in the Northeastern Atlantic, the Mediterranean and Connected Seas (NEAMTWS), Version 2.00, https://www.ingv.it/cat/images/images/documenti/NEAMTWS-OpUG-version_2_0_Final.pdf (last access: 18 June 2022), 2012a.
UNESCO/IOC: Global Sea Level Observing System (GLOSS) Implementation Plan, 2012b.
UNESCO/IOC: Quality Control of in situ sea level observations: a review and progress towards automated quality control, Vol. 1, UNESCO IOC Manuals and Guides No. 83 (IOC/2020/MG/83 Vol. 1), 2020.
Vela, J., Pérez Gómez, B., González, M., Otero, L., Olabarrieta, M., Canals, M., and Casamor, J. L.: Tsunami resonance in Palma Bay and harbor, Majorca Island, as induced by the 2003 Western Mediterranean earthquake, J. Geol., 122, 165–182, https://doi.org/10.1086/675256, 2014.
Vignudelli, S., Birol, F., Benveniste, J., Fu, L.L., Picot, N., Raynal, M., and Roinard, H.: Satellite altimetry measurements of sea level in the coastal zone, Surv. Geophys., 40, 1319–1349, https://doi.org/10.1007/s10712-019-09569-1, 2019.
Vilibić, I., Orlić, M., Čupić, S., Domijan, N., Leder, N., Mihanović, H., Pasarić, M., Pasarić, Z., Srdelić, M., and Strinić, G.: A new approach to sea level observations in Croatia, Geofizika, 22, 21–57, 2005.
Vilibić, I., Monserrat, S., Rabinovich, A. B., and Mihanović, H.: Numerical modelling of the destructive meteotsunami of 15 June 2006 on the coast of the Balearic Islands, Pure Appl. Geophys., 165, 2169–2195, https://doi.org/10.1007/s00024-008-0426-5, 2008.
Vilibić, I., Šepić, J., Ranguelov, B., Strelec Mahović, N., and Tinti, S.: Possible atmospheric origin of the 7 May 2007 western Black Sea shelf tsunami event, J. Geophys. Res., 115, C07006, https://doi.org/10.1029/2009JC005904, 2010.
Vilibić, I., Šepić, J., Rabinovich, A. B., and Monserrat, S.: Modern approaches in meteotsunami research and early warning, Front. Mar. Sci., 3, 57, https://doi.org/10.3389/fmars.2016.00057, 2016.
Vilibić, I., Denamiel, C., Zemunik, P., and Monserrat, S.: The Mediterranean and the Black Sea meteotsunamis: an overview, Nat. Hazards, 106, 1223–1267, https://doi.org/10.1007/s11069-020-04306-z, 2021.
Wahl, T. and Chambers, D. P.: Evidence for multidecadal variability in US extreme sea level records, J. Geophys. Res.-Oceans, 120, 1527–1544, https://doi.org/10.1002/2014JC010443, 2015.
Wilkinson, M. D., Dumontier, M., Aalbersberg, I. J., Appleton, G., Axton, M., Baak, A., Blomberg, N., Boiten, J.-W., da Silva Santos, L. B., Bourne, P. E., Bouwman, J., Brookes, A. J., Clark, T., Crosas, M., Dillo, I., Dumon, O., Edmunds, S., Evelo, C. T., Finkers, R., Gonzalez-Beltran, A., Gray, A. J. G., Groth, P., Goble, C., Grethe, J. S., Heringa, J., 't Hoen, P. A. C., Hooft, R., Kuhn, T., Kok, R., Kok, J., Lusher, S. J., Martone, M. E., Mons, A., Packer, A. L., Persson, B., Rocca-Serra, P., Roos, M., van Schaik, R., Sansone, S.-A., Schultes, E., Sengstag, T., Slater, T., Strawn, G., Swertz, M. A., Thompson, M., van der Lei, J., van Mulligen, E., Velterop, J., Waagmeester, A., Wittenburg, P., Wolstencroft, K., Zhao, J., and Mons, B.: The FAIR Guiding Principles for scientific data management and stewardship, Sci. Data, 3, 160018, https://doi.org/10.1038/sdata.2016.18, 2016.
Woodworth, P. L. and Player, R.: The Permanent Service for Mean Sea Level: An update to the 21st century, J. Coastal Res., 19, 287–295, 2003.
Woodworth, P. L., Rickards, L. J., and Pérez, B.: A survey of European sea level infrastructure, Nat. Hazards Earth Syst. Sci., 9, 927–934, https://doi.org/10.5194/nhess-9-927-2009, 2009.
Woodworth, P. L., Hunter, J. R., Marcos Moreno, M., Caldwell, P. C., Menendez, M., and Haigh, I. D.: GESLA (Global Extreme Sea Level Analysis) high frequency sea level dataset – Version 2, British Oceanographic Data Centre – Natural Environment Research Council, UK, https://doi.org/10.5285/3b602f74-8374-1e90-e053-6c86abc08d39, 2016.
Woodworth, P. L., Hunter, J. R., Marcos, M., Caldwell, P., Menéndez, M., and Haigh, I.: Towards a global higher-frequency sea level dataset, Geosci. Data J., 3, 50–59, https://doi.org/10.1002/gdj3.42, 2017.
Woodworth, P. L., Wöppelmann, G., Marcos, M., Gravelle, M., and Bingley, R. M.: Why we must tie satellite positioning to tide gauge data, Eos, 98, https://doi.org/10.1029/2017EO064037, 2017.
Woodworth, P. L., Melet, A., Marcos, M., Ray, R. D., Wöppelmann, G., Sasaki, Y. N., Cirano, M., Hibbert, A., Huthnance, J. M., Monserrat, S., and Merrifield, M. : Forcing factors affecting sea level changes at the coast, Surv. Geophys., 40, 1351–1397, https://doi.org/10.1007/s10712-019-09531-1, 2019.
Wöppelmann, G., Marcos, M., Coulomb, A., Martín Míguez, B., Bonnetain, P., Boucher, C., Gravelle, M., Simon, B., and Tiphaneau, P.: Rescue of the historical sea level record of Marseille (France) from 1885 to 1988 and its extension back to 1849–1851, J. Geodesy, 88, 869–885, https://doi.org/10.1007/s00190-014-0728-6, 2014.
Yang, Y., Jin, T., Gao, X., Wen, H., Schöne, T., Xiao, M., and Huang, H.: Sea level fusion of satellite altimetry and tide gauge data by deep learning in the Mediterranean Sea, Remote Sens., 13, 908, https://doi.org/10.3390/rs13050908, 2021.
Zanchettin, D., Bruni, S., Raicich, F., Lionello, P., Adloff, F., Androsov, A., Antonioli, F., Artale, V., Carminati, E., Ferrarin, C., Fofonova, V., Nicholls, R. J., Rubinetti, S., Rubino, A., Sannino, G., Spada, G., Thiéblemont, R., Tsimplis, M., Umgiesser, G., Vignudelli, S., Wöppelmann, G., and Zerbini, S.: Sea-level rise in Venice: historic and future trends (review article), Nat. Hazards Earth Syst. Sci., 21, 2643–2678, https://doi.org/10.5194/nhess-21-2643-2021, 2021.
Zemunik, P., Bonanno, A., Mazzola, S., Giacalone, G., Fontana, I., Genovese, S., Basilone, G., Candela, J., Šepić, J., Vilibić, I., and Aronica, S.: Observing meteotsunamis (“Marrobbio”) in the southwestern coast of Sicily, Nat. Hazards, 106, 1337–1363, https://doi.org/10.1007/s11069-020-04303-2, 2021a.
Zemunik, P., Šepić, J., Pellikka, H., Ćatipović, L., and Vilibić, I.: Minute Sea-Level Analysis (MISELA): a high-frequency sea-level analysis global dataset, Earth Syst. Sci. Data, 13, 4121–4132, https://doi.org/10.5194/essd-13-4121-2021, 2021b.
Zerbini S., Plag, H.-P., Baker, T., Becker, M., Billiris, H., Bürki, B., Kahle, H.-G., Marson, I., Pezzoli, L., Richter, B., Romagnoli, C., Sztobryn, M., Tomasi, P., Tsimplis, M., Veis, G., and Verrone, G.: Sea level: in the Mediterranean: a first step towards separating crustal movements and absolute sea-level variations, Global Planet. Change, 14, 1–48, https://doi.org/10.1016/0921-8181(96)00003-3, 1996.
Zerbini, S., Raicich, F., Prati, C. M., Bruni, S., Del Conte, S., Errico, M., and Santi, E.: Sea-level change in the Northern Mediterranean Sea from long-period tide gauge time series, Earth-Sci. Rev., 167, 72–87, https://doi.org/10.1016/j.earscirev.2017.02.009, 2017.
Zhuk, E., Zodiatis, G., and Sylaios, G.: The Web GIS monitoring and forecasting platforms in the Mediterranean and Black seas, Proc. SPIE 11524, Eighth International Conference on Remote Sensing and Geoinformation of the Environment (RSCy2020), 359–365, 26 August 2020, https://doi.org/10.1117/12.2570969, 2020.
Žust, L., Fettich, A., Kristan, M., and Ličer, M.: HIDRA 1.0: deep-learning-based ensemble sea level forecasting in the northern Adriatic, Geosci. Model Dev., 14, 2057–2074, https://doi.org/10.5194/gmd-14-2057-2021, 2021.
- Abstract
- Introduction
- Tide gauge networks and observations
- Data availability in existing international programmes
- Assessment of the network to fulfil targeted applications
- Discussion and conclusions
- Appendix A
- Data availability
- Author contributions
- Competing interests
- Disclaimer
- Special issue statement
- Acknowledgements
- Financial support
- Review statement
- References
- Supplement
- Article
(8424 KB) - Full-text XML
- Abstract
- Introduction
- Tide gauge networks and observations
- Data availability in existing international programmes
- Assessment of the network to fulfil targeted applications
- Discussion and conclusions
- Appendix A
- Data availability
- Author contributions
- Competing interests
- Disclaimer
- Special issue statement
- Acknowledgements
- Financial support
- Review statement
- References
- Supplement