the Creative Commons Attribution 4.0 License.
the Creative Commons Attribution 4.0 License.
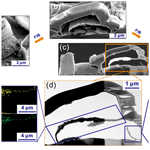
Occurrence of structural aluminium (Al) in marine diatom biological silica: visible evidence from microscopic analysis
Qian Tian
Peng Yuan
Mengyuan Li
Weifeng Yang
Jieyu Zhou
Huihuang Wei
Junming Zhou
Haozhe Guo
The global marine biogeochemical cycle of aluminium (Al) is believed to be driven by marine diatoms, due to the uptake of dissolved Al (DAl) by living diatoms from surface seawater. The occurrence of Al in diatom biogenic silica (BSi) can inhibit the dissolution of BSi, thus benefiting the effects of the ballast role of diatoms in the biological pump and forming a coupled Si–Al biogeochemical cycle. However, the occurrence characteristic of Al in marine diatoms is still unclear. In particular, whether or not Al is incorporated into the structure of BSi of living diatoms is unrevealed, resulting in difficulties in understanding the biogeochemical behaviours of Al. In this study, Thalassiosira weissflogii, a widely distributed marine diatom in marginal seas, was selected as the model to evaluate the occurrence of structural Al in BSi based on culturing experiments with the addition of DAl. The structural Al in BSi was detected by combining focused ion beam (FIB) scanning electron microscopy and energy-dispersive X-ray spectroscopy (EDS) mapping analysis. Visible, direct evidence of structural Al in living BSi was obtained, and the distribution and content of this Al were revealed by the EDS-mapping analysis. The effects of structural Al on BSi dissolution–inhibition are discussed based on the content of this Al. The fundamental results indicate the significant contribution of marine diatoms to the biogeochemical migration of marine Al.
- Article
(6036 KB) - Full-text XML
-
Supplement
(261 KB) - BibTeX
- EndNote
Aluminium (Al) is the most abundant metal element in the Earth's crust, and it mainly occurs in Al-bearing minerals such as aluminosilicate (Taylor, 1964). The weathering of such aluminosilicates results in a huge carbon (C) sink in rocks and soils due to the transformation of CO2 to HCO based on the reaction with such Al-bearing minerals (Mackenzie and Kump, 1995; Wallmann et al., 2008). Therefore, Al is involved in the terrestrial C cycle (Stallard, 1998). Despite the high-Al content of the terrestrial system, the concentration of dissolved Al (DAl) in natural water is very low (from <1.0 nM to more than 100.0 nM in oceans; up to several micromolars in river mouths) (Hydes, 1977; Measures and Edmond, 1990; van Beusekom et al., 1991; Chou and Wollast, 1997; Gillmore et al., 2016; Menzel Barraqueta et al., 2020). Thus, oceanic DAl is often used as a tracer for the transportation of terrestrial substances and the mixing of different water masses (Measures and Vink, 1999, 2000; Han et al., 2008; Schlitzer et al., 2018).
Due to its lack of an essential biological role (even toxicity) (Xie et al., 2015; Gillmore et al., 2016), the terrestrial cycling of Al is believed to be primarily controlled by inorganic processes. However, marine diatoms are found to take up and incorporate Al into their cells (Q. Liu et al., 2019). Diatoms can eliminate plenty of DAl from surface water when they bloom (Hall et al., 1999; Ren et al., 2011), and they export Al into the sedimentary layers through the sedimentation of post-mortem diatoms, forming a coupled Si–Al biogeochemical cycle in the oceans (van Hulten et al., 2014).
Diatoms are a type of widely distributed single-cell algae in the oceans, and they account for up to ∼ 40 % of the oceanic primary production (Armbrust, 2009). Through sedimentation of dead diatoms, huge amounts of organic carbon (OC) are exported from the surface ocean to the deep ocean (Nelson et al., 1996; Riebesell, 2000; Leblanc et al., 2018) controlling the oceanic C cycle and forming an important part of the biological carbon pump (Smetacek, 1999; Tréguer and Pondaven, 2000; Ragueneau et al., 2006).
Compared with other marine phytoplankton, diatoms are more effective at carbon sequestration over longer timescales due to their frustules, which are composed of biogenic silica (BSi) with high mechanical stability (Baines et al., 2010). Since it acts as a ballast (De La Rocha et al., 2008; Honda and Watanabe, 2010), BSi carries the OC to deeper oceans and deposits on the seafloor, forming a coupled Si–C cycle.
Considering the key role of diatoms in carbon sequestration, the Al in diatom cells is also involved in the C cycle in the oceans, and thus, Al participates in the global C geochemical cycle through inorganic and biological processes (Gehlen et al., 2002, 2003; Ren et al., 2013). Although preliminary studies have been carried out to investigate the Al in freshwater (D. Liu et al., 2019) and marine diatoms, the occurrence of Al in marine diatoms is far away unclear, which results in difficulties in understanding the abovementioned diatom-derived Al cycle.
That Al is incorporated into the organic components, and BSi of diatoms has been studied. For organic components, the occurrence mechanism and distribution have been well described (Q. Liu et al., 2019). For BSi of marine diatoms, the coordination of Al in BSi was identified by using X-ray absorption spectrometry (XAS) and nuclear magnetic resonance (NMR) spectroscopy, showing Al with tetrahedral coordination (Beck et al., 2002; Gehlen et al., 2002; Koning et al., 2007; Machill et al., 2013). However, the information of this Al, including its content and distribution characteristics, is lacking. Moreover, the previous evaluation cannot avoid the interference of the Al from organic components of diatoms and Al-bearing minerals. For the former, the high activity of fresh BSi results in the easy adsorption of DAl from Al-containing solutions during the removal of the Al-bearing organic components of diatoms, which interferes with the evaluation of the structural Al (Moran and Moore, 1988; Koning et al., 2007). For the latter, Al-bearing tiny mineral particles can hardly be excluded from sedimentary BSi even with the greatest of care, e.g. the acid washing reported by Gehlen et al. (2002). Due to these challenges, the characteristics of Al in the marine BSi structure are still unclear, although possible contents and coordinated states have been proposed. The reason that so much attention has been paid to the structural Al in BSi is that structural Al has a dissolution–inhibition effect on BSi. About 25 % decrease in the solubility of BSi was shown when Al substitutes for 1 out of every 70 Si atoms (Dixit et al., 2001), and thus, structural Al is believed to be one of the key factors that influence the transfer of BSi from the surface ocean to pelagic sediments. Therefore, the indefinite occurrence characteristics of Al in BSi severely limit our understanding of the composition, structure, and water stability of BSi.
In this study, a widely distributed marine diatom in marginal seas, Thalassiosira weissflogii (T. weissflogii) (Panagiotopoulos et al., 2020), was selected as the model; culture experiments were performed with the addition of high concentrations of DAl. The direct detection of the internal surface of BSi was conducted using a focused ion beam (FIB) pretreatment, which can remove the external surface, leading to exposing the internal surface of BSi. Energy-dispersive X-ray spectroscopy (EDS) mapping analysis was used to determine the content and distribution of the structural Al (Twining et al., 2008). Finally, the effects of structural Al on the BSi dissolution and the carbon sequestration efficiency based on the coupled Si–Al cycle controlled by marine diatoms were discussed.
2.1 Cultivation of the diatoms
T. weissflogii, purchased from the Shanghai Guangyu Biological Technology Co., Ltd (China), was used as the model diatom in this study to investigate Al occurrence. The species of the marine diatom samples were identified by determining their rbcL gene sequences (Couradeau et al., 2012). The obtained phylogram clearly showed that the diatoms obtained were T. weissflogii (Fig. 1).
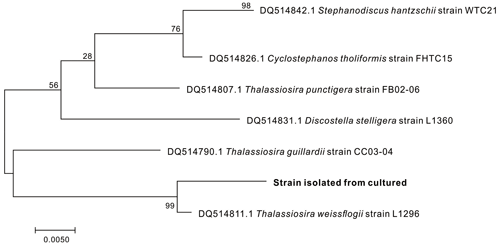
Figure 1Phylogenetic trees of diatom strains isolated from the culturing diatoms. The scale bar indicates the number of substitutions per site for a unit branch length.
The diatoms were cultured in artificial seawater supplemented with medium at 25 ∘C under a light dark cycle at an intensity of 100 µmol photons m−2 s−2 for 14 d. The composition of artificial seawater is presented in Table S1 (see Supplement), and culture medium was prepared following the previous report (Guillard, 1975; Guillard and Ryther, 1962). Deionized water was used to prepare the artificial seawater and medium. The initial concentration of DAl in culture medium without the addition of Al was several nanomolars. Cell density of the diatoms in the culture media with/without the addition of Al were counted using flow cytometry (BioMarine-IA 1000, Countstar Company, Shanghai, China).
Trace-metal clean processes were used to eliminate the interference from other elements. Before the cultivation experiment, all of the bottles and tubes were soaked in 10 % hydrochloric acid for 24 h, sterilized at 120 ∘C for 30 min, and washed repeatedly with deionized water (Q. Liu et al., 2019).
AlCl3 (i.e. the Al source) was added to the culture medium with an initial concentration of 2.0 µM in the medium. The control experiment was also simultaneously performed without adding AlCl3. The pH value of the diatom culture medium was adjusted to 8.0 using NaOH solution. All of the chemical reagents were allowed to stand overnight to reach chemical equilibrium before use.
2.2 Collection of diatoms and extraction of diatom frustules
After 14 d of culturing, the diatoms were collected through high-speed centrifugation at 11 000 rpm. The obtained diatoms (Fig. 2) were rinsed with deionized water three times to remove any impurities adsorbed on the surface. The solid obtained was freeze-dried after centrifugation. Two pretreatment procedures were used to remove any Al adsorbed on the surface of the diatoms and their organic components to obtain pure BSi: (1) immersion in 0.05 M EDTA for 12 h followed by washing three times and (2) immersion in 30 % hydrogen peroxide solution for 48 h followed by washing five times. The removal of the organic components was confirmed using a Vario EL III elemental analyser with a detection limit of <40 ppm. Approximately 2.00 mg of sample was placed in a 6 × 12 mm tin boat and was oxidized in a combustion tube in the presence of oxygen at high temperature (≥ 1150 ∘C).
2.3 Characterization and data analysis
2.3.1 Focused ion beam (FIB) treatment and characterization methods
The FIB milling was carried out using FIB scanning electron microscopy (SEM; FEI Helios Nano Lab 450S) equipped with a flip stage, an in situ scanning transmission electron microscope (STEM) detector, a Tomahawk ion column, and a multichannel gas injection system. For the FIB milling conducted to obtain a thin BSi slice, a single frustule of BSi selected for characterization was picked using a nanomanipulator (Oxford OmniProbe 400), and a 5 kV focused gallium ion (Ga+) beam with a beam current of 40 pA was used with the total milling time of 2 min (Schematic representation of the FIB-milling processes, see Supplement Fig. S1). Then, the obtained BSi slices (with a thickness of approximately 80 nm) were fixed on the edge of the FIB half grid through induced platinum (Pt) deposition. The in situ field emission SEM (FE-SEM) observation of the BSi slice was performed with an accelerating voltage of 30 kV and a current of 24–9300 pA. The elemental distribution of the sliced BSi was obtained using an energy-dispersive X-ray spectrometer attached to a transmission electron microscope (FEI Talos F200 TEM/EDS microscope) with a voltage of 200 kV.
2.3.2 Energy-dispersive spectroscopy (EDS) spot analysis
In previous studies, the change in the Al concentrations of the culture medium was used to evaluate the Al uptake of diatoms. However, some of the Al forms a new Al-bearing phase based on changes in the chemical environment (such as reverse weathering) (Isson and Planavsky, 2018), rather than being involved in the biological processes of diatoms. To avoid the disturbance of such Al, which does not incorporate into the cells of the diatoms, the atomic ratios of the cultured diatoms and their BSi were determined using EDS spot analysis and a scanning electron microscope (SU8010, UltraPore-300, PDP-200). More than 300 spots from 35 whole diatoms and the BSi of the T. weissflogii were detected (details see Supplement), and the atomic ratios were obtained at a voltage of 15 kV and a current of 20 µA. Carbon coating was performed before the SEM-EDS analysis.
The data for the specific growth rate and EDS spot analysis were obtained on the basis of the mean ± standard deviation (SD; n≥3). One-way analysis of variance (ANOVA) was used to determine the significant differences (p<0.05) between the treatments (Shi et al., 2015).
3.1 Effects of Al on the growth of diatoms
To investigate the influence of Al on the growth of T. weissflogii, the cell density was measured during the diatom cultivation processes. The stable growth period of the diatoms cultured with and without the addition of Al began on the third day. The growth trends of the diatom cells were similar in the diatom culture mediums with and without the addition of Al (Fig. 3). The final concentration of the diatom cells exhibited a very slight difference, with average values of 1.96 × 105 and 1.91 × 105 cells mL−1 for the Al-free diatom culture medium and the Al-added medium, respectively. The results indicate that the presence of Al did not significantly affect the cell yields of T. weissflogii during the 14 d culture period.
3.2 Identification of structural Al in BSi based on FIB-EDS
FIB milling can remove the external surface of the diatom and impurities adsorbed onto it, allowing for the detection of the inner structure of the BSi. Thus, the subsequent EDS detection obtains the structural element distributions of the BSi. In this case, the combined FIB–EDS analyses could detect the structural elements of BSi and avoid the disturbance of the non-structural elements (those adsorbed on the external surface of BSi). The T. weissflogii BSi sample was selected (Fig. 4a and the inset) and milled to a thin slice using FIB (Fig. 4b and c). Two inner areas of the BSi slice were observed (blue rectangles in Fig. 4d), and the Al distribution was detected in these two domains, revealing a similar distribution characteristic of Al to Si in the different regions of BSi structure; that is, the higher the Si concentration is, the higher the Al concentration is (Fig. 4e and g).
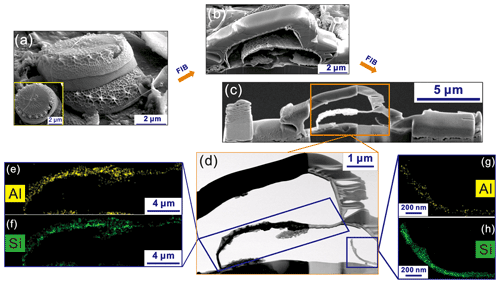
Figure 4(a and the inset) FESEM image of a T. weissflogii BSi; (b, c) FESEM images of a series of FIB milling products of the BSi during the ion sputtering experiments; (d) HRTEM bright field (BF) image of the selected domain in the rectangle in (c); (e, g) Al and (f, h) Si distributions in the domains in (d) obtained using EDS mapping analysis.
This is direct evidence of the presence of Al in the internal surface of BSi sourced from living marine diatoms, demonstrating the occurrence of structural Al in the diatomaceous biological framework of marine diatoms. The Al is incorporated into the BSi and used to build diatom BSi. Based on the EDS mapping analysis, the average atomic ratio is 0.011.
3.3 Quantity measurement of the Al in the diatoms and their BSi using EDS spot analysis
More than 300 spots on diatoms and their BSi were analysed using EDS, and the average atomic ratios were 0.086 and 0.030 for the entire diatoms and their BSi, respectively. The value of the BSi is lower than that of the entire diatoms (Fig. 5), because Al occurs not only in BSi but also in the organic components of the diatoms. The Al content of the entire diatom is nearly 3-fold higher than that of the BSi. Moreover, the average atomic ratio of the BSi (0.030) obtained through EDS spot analysis is higher than that obtained through FIB-EDS mapping (0.011).
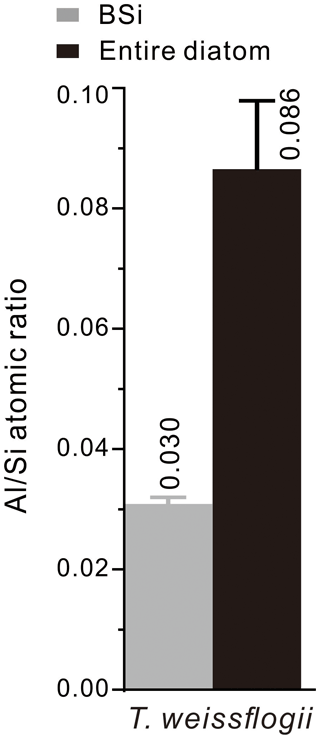
Figure 5 atomic ratios of the T. weissflogii diatom cells (the black column) cultured in media with the addition of Al and of their BSi (the grey column). The EDS spot analysis used to determine the atomic ratios was based on more than 300 spots on 35 diatom cells and their BSi samples. The error bars were obtained through mean ± standard deviation analysis of all of the atomic ratios.
The scavenging of Al from seawater by diatoms has been widely observed during diatom blooms (Hall et al., 1999; Ren et al., 2011). This unique behaviour of Al uptake has attracted a great deal of attention, and a corresponding biogeochemical Al cycle driven by diatoms was hypothesized (van Hulten et al., 2014). Proving the occurrence of Al in diatoms is key to understanding the mechanism of Al uptake by marine diatoms. Q. Liu et al. (2019) investigated Al incorporation into diatoms and proposed the distribution and the content of Al in the organic components of diatoms based on various extraction methods (Q. Liu et al., 2019). Occurrence of Al in BSi has been investigated (Beck et al., 2002; Gehlen et al., 2002; Koning et al., 2007; Machill et al., 2013). The coordination characteristic of Al in BSi which was obtained from living diatoms and sediments was evaluated using the Al K-edge X-ray absorption near-edge structure (XANES) spectroscopy and NMR spectroscopy (Beck et al., 2002; Gehlen et al., 2002; Koning et al., 2007), showing Al with tetrahedral coordination in BSi. Therefore, the coupled Si and Al biogeochemical cycle in oceans was proposed based on these results. However, the distribution and content of structural Al in BSi of marine diatoms was still not well-known.
During the evaluation of Al in BSi, avoiding the interference of the Al in organic components of diatoms and in Al-bearing minerals loading on the surface of BSi in sediments is very difficult. Removing organic components of diatoms could release Al, and some Al would be adsorbed by the fresh BSi which possesses the high activity (Koning et al., 2007). Moreover, sedimentary BSi contained plenty of Al-bearing tiny mineral particles which are hardly removed by physical and chemical pre-treatments (Michalopoulos et al., 2000; Gehlen et al., 2002; Koning et al., 2007). The two types of Al influence the detection of the Al incorporated in the BSi, preventing the identification and quantification of the structural Al. Due to these problems, the Al detected through elemental analyses such as X-ray fluorescence (XRF), and inductively coupled plasma optical emission spectroscopy (ICP-OES) for the DAl in diatom culture medium (de Jonge et al., 2010; Machill et al., 2013), and EDS for marine BSi is difficult to evaluate structural Al content and even to identify its occurrence.
In our previous study, we found that FIB milling allowed us to obtain a slice of an internal section of BSi and to remove the external surface, avoiding the disturbance of any impurities in the further detection of the structural elements (D. Liu et al., 2019). The freshwater BSi was investigated to reveal Al occurring in the structure of BSi and a high-level concentration of Al was detected. Through a combination of FIB and EDS, direct and visible evidence was obtained, illustrating the distribution and quantity of the structural Al in BSi. Structural Al was observed in BSi of marine diatoms, demonstrating that marine diatoms take up Al and use it to build their siliceous framework (BSi). Similar to freshwater diatom BSi, Al shows similar distribution characteristics to Si in marine BSi, but the Al content of marine diatoms ( atomic ratio of ∼ 0.011) is much lower than that of freshwater diatoms (∼ 0.050) based on the atomic ratio obtained by FIB-EDS analysis (D. Liu et al., 2019; Yuan et al., 2019). However, the value is slightly higher than the maximum (0.008) reported in previous studies (Gehlen et al., 2002; Koning et al., 2007). This may be due to the high Al concentration of the culture medium (more than up to 1000-fold over the Al concentration in the seawater of the open ocean) (Han et al., 2008) and the type of the diatoms which have various levels of silicification, probably affecting the ratio. It should be noted that the atomic ratio of BSi, which was extracted from living diatoms by removing the organic components of the diatoms, is much larger than the value obtained by FIB-EDS. This result may be due to the disturbance of non-structural Al, which is sourced from the deconstruction of the organic components of the diatoms or loosely bound aluminium and is subsequently adsorbed onto the surface of BSi. The Al that occurs in the BSi structure was deposited with the BSi, and thus the BSi in sediments constitutes a diatom-driven biological Al sink.
Moreover, structural Al is proposed to have a dissolution–inhibition effect on BSi, i.e. a 25 % decrease in BSi solubility when Al substitutes for 1 out of every 70 Si atoms ( atomic ratio of 0.014) (Dixit et al., 2001; van Cappellen et al., 2002). Since the atomic ratio of the BSi structure is 0.011 (obtained by FIB-EDS analysis), the BSi solubility will be decreased by ∼ 20 %, based on the relationship between Al content and BSi dissolution–inhibition. Therefore, this structural Al makes a high contribution to BSi burial. Considering that a huge quantity of carbon settles into the sedimentary layers every year based on BSi exports (Smetacek, 1999; Tréguer et al., 2017), the dissolution–inhibition effect of structural Al on BSi may influence or even control the efficiency of the carbon sequestration of the diatom-driven biological pump.
In this study, the occurrence characteristics of structural Al in BSi of marine diatoms, T. weissflogii, were investigated using a combination of FIB pretreatment and SEM-EDS analyses. Direct, visible evidence of the presence of structural Al in living BSi and its characteristic including distribution and content were obtained based on the FIB-EDS mapping analysis of BSi slices. The average atomic ratio in the structure of the BSi was 0.011, and thus a high contribution of the structural Al to the dissolution–inhibition of the BSi was proposed. Considering the influence of structural Al on the solubility of diatom BSi, the occurrence of structural Al in BSi can influence the carbon sequestration efficiency of the diatom-driven biological pump.
The data generated for this study are available on request to the corresponding author.
The supplement related to this article is available online at: https://doi.org/10.5194/os-18-321-2022-supplement.
QT and DL designed the experiments and wrote the manuscript. HW and ML were collected the samples of marine diatoms. QT and JZ carried out the culture of diatoms and EDS analysis. PY and WY were involved in the discussion on the data analysis and revision of the draft. DL, JZ, and HG were involved in the FIB analysis.
The contact author has declared that neither they nor their co-authors have any competing interests.
Publisher’s note: Copernicus Publications remains neutral with regard to jurisdictional claims in published maps and institutional affiliations.
This work is supported by the National Natural Scientific Foundation of China (grant nos. 42172047 and 41772041), the funding of State Key Laboratory of Marine Environmental Science (MEL) Visiting Fellowship of Xiamen University (MELRS2006 grant), Jiangxi Province technology innovation guidance project (grant no. 20212BDH81036), and Science and Technology Planning Project of Guangdong Province (grant no. 2020B1212060055). This is a contribution (grant no. IS-3134) from GIGCAS.
This research has been supported by the National Natural Scientific Foundation of China (grant nos. 42172047 and 41772041), the funding of State Key Laboratory of Marine Environmental Science (MEL) Visiting Fellowship of Xiamen University (MELRS2006 grant), Jiangxi Province technology innovation guidance project (grant no. 20212BDH81036), and Science and Technology Planning Project of Guangdong Province (grant no. 2020B1212060055).
This paper was edited by Anne Marie Tréguier and reviewed by two anonymous referees.
Armbrust, E. V.: The life of diatoms in the world's oceans, Nature, 459, 185–192, https://doi.org/10.1038/nature08057, 2009.
Baines, S. B., Twining, B. S., Brzezinski, M. A., Nelson, D. M., and Fisher, N. S.: Causes and biogeochemical implications of regional differences in silicification of marine diatoms, Global Biogeochem. Cy., 24, 1–15, https://doi.org/10.1029/2010gb003856, 2010.
Beck, L., Gehlen, M., Flank, A. M., van Bennekom, A. J., and van Beusekom, J. E. E.: The relationship between Al and Si in biogenic silica as determined by PIXE and XAS, Nucl. Instrum. Meth. B, 189, 180–184, https://doi.org/10.1016/S0168-583X(01)01035-7, 2002.
Chou, L. and Wollast, R.: Biogeochemical behavior and mass balance of dissolved aluminum in the western Mediterranean Sea, Deep-Sea Res. Pt. II, 44, 741–768, https://doi.org/10.1016/s0967-0645(96)00092-6, 1997.
Couradeau, E., Benzerara, K., Gerard, E., Moreira, D., Bernard, S., Brown Jr., G. E., and Lopez-Garcia, P.: An Early-Branching Microbialite Cyanobacterium Forms Intracellular Carbonates, Science, 336, 459–462, https://doi.org/10.1126/science.1216171, 2012.
de Jonge, M. D., Holzner, C., Baines, S. B., Twining, B. S., Ignatyev, K., Diaz, J., Howard, D. L., Legnini, D., Miceli, A., McNulty, I., Jacobsen, C. J., and Vogt, S.: Quantitative 3D elemental microtomography of Cyclotella meneghiniana at 400-nm resolution, P. Natl. Acad. Sci. USA, 107, 15676–15680, https://doi.org/10.1073/pnas.1001469107, 2010.
De La Rocha, C. L., Nowald, N., and Passow, U.: Interactions between diatom aggregates, minerals, particulate organic carbon, and dissolved organic matter: Further implications for the ballast hypothesis, Global Biogeochem. Cy., 22, GB4005, https://doi.org/10.1029/2007gb003156, 2008.
Dixit, S., van Cappellen, P., and van Bennekom, A. J.: Processes controlling solubility of biogenic silica and pore water build-up of silicic acid in marine sediments, Mar. Chem., 73, 333–352, https://doi.org/10.1016/s0304-4203(00)00118-3, 2001.
Gehlen, M., Beck, L., Calas, G., Flank, A. M., van Bennekom, A. J., and van Beusekom, J. E. E.: Unraveling the atomic structure of biogenic silica: Evidence of the structural association of Al and Si in diatom frustules, Geochim. Cosmochim. Ac., 66, 1601–1609, https://doi.org/10.1016/s0016-7037(01)00877-8, 2002.
Gehlen, M., Heinze, C., Maier-Reimer, E., and Measures, C. I.: Coupled Al-Si geochemistry in an ocean general circulation model: A tool for the validation of oceanic dust deposition fields?, Global Biogeochem. Cy., 17, 1028, https://doi.org/10.1029/2001GB001549, 2003.
Gillmore, M. L., Golding, L. A., Angel, B. M., Adams, M. S., and Jolley, D. F.: Toxicity of dissolved and precipitated aluminium to marine diatoms, Aquat. Toxicol., 174, 82–91, https://doi.org/10.1016/j.aquatox.2016.02.004, 2016.
Guillard, R. R. and Ryther, J. H.: Studies of marine planktonic diatoms, I. Cyclotella nana Hustedt, and Detonula confervacea (cleve) Gran, Can. J. Microbiol., 8, 229–239, https://doi.org/10.1139/m62-029, 1962.
Guillard, R. R. L.: Culture of phytoplankton for feeding marine invertebrates, in: culture of marine invertebrate animals: proceedings – 1st Conference on Culture of Marine Invertebrate Animals Greenport, edited by: Smith, W. L. and Chanley, M. H., Springer US, Boston, MA, 29–60, https://doi.org/10.1007/978-1-4615-8714-9_3, 1975.
Hall, I. R., Hydes, D. J., Statham, P. J., and Overnell, J.: Seasonal variations in the cycling of aluminium, cadmium and manganese in a Scottish sea loch: biogeochemical processes involving suspended particles, Cont. Shelf Res., 19, 1783–1808, https://doi.org/10.1016/s0278-4343(99)00056-4, 1999.
Han, Q., Moore, J. K., Zender, C., Measures, C., and Hydes, D.: Constraining oceanic dust deposition using surface ocean dissolved Al, Global Biogeochem. Cy., 22, GB2003, https://doi.org/10.1029/2007GB002975, 2008.
Honda, M. C. and Watanabe, S.: Importance of biogenic opal as ballast of particulate organic carbon (POC) transport and existence of mineral ballast-associated and residual POC in the Western Pacific Subarctic Gyre, Geophys. Res. Lett., 37, L02605, https://doi.org/10.1029/2009GL041521, 2010.
Hydes, D. J.: Dissolved aluminium concentration in sea water, Nature, 268, 136–137, https://doi.org/10.1038/268136a0, 1977.
Isson, T. T. and Planavsky, N. J.: Reverse weathering as a long-term stabilizer of marine pH and planetary climate, Nature, 560, 471–475, https://doi.org/10.1038/s41586-018-0408-4, 2018.
Koning, E., Gehlen, M., Flank, A. M., Calas, G., and Epping, E.: Rapid post-mortem incorporation of aluminum in diatom frustules: Evidence from chemical and structural analyses, Mar. Chem., 106, 208–222, https://doi.org/10.1016/j.marchem.2006.06.009, 2007.
Leblanc, K., Quéguiner, B., Diaz, F., Cornet, V., Michel-Rodriguez, M., Durrieu de Madron, X., Bowler, C., Malviya, S., Thyssen, M., Grégori, G., Rembauville, M., Grosso, O., Poulain, J., de Vargas, C., Pujo-Pay, M., and Conan, P.: Nanoplanktonic diatoms are globally overlooked but play a role in spring blooms and carbon export, Nat. Commun., 9, 953, https://doi.org/10.1038/s41467-018-03376-9, 2018.
Liu, D., Yuan, P., Tian, Q., Liu, H., Deng, L., Song, Y., Zhou, J., Losic, D., Zhou, J., Song, H., Guo, H., and Fan, W.: Lake sedimentary biogenic silica from diatoms constitutes a significant global sink for aluminium, Nat. Commun., 10, 4829, https://doi.org/10.1038/s41467-019-12828-9, 2019.
Liu, Q., Zhou, L., Liu, F., Fortin, C., Tan, Y., Huang, L., and Campbell, P. G. C.: Uptake and subcellular distribution of aluminum in a marine diatom, Ecotox. Environ. Safe., 169, 85–92, https://doi.org/10.1016/j.ecoenv.2018.10.095, 2019.
Machill, S., Kohler, L., Ueberlein, S., Hedrich, R., Kunaschk, M., Paasch, S., Schulze, R., and Brunner, E.: Analytical studies on the incorporation of aluminium in the cell walls of the marine diatom Stephanopyxis turris, Biometals, 26, 141–150, https://doi.org/10.1007/s10534-012-9601-3, 2013.
Mackenzie, F. T. and Kump, L. R.: Reverse weathering, clay mineral formation, and oceanic element cycles, Science, 270, 586–587, https://doi.org/10.1126/science.270.5236.586, 1995.
Measures, C. I. and Edmond, J. M.: Aluminum in the south-atlantic – steady-state distribution of a short residence time element, J. Geophys. Res.-Ocean., 95, 5331–5340, https://doi.org/10.1029/JC095iC04p05331, 1990.
Measures, C. I. and Vink, S.: Seasonal variations in the distribution of Fe and Al in the surface waters of the Arabian Sea, Deep-Sea Res. Pt. II, 46, 1597–1622, https://doi.org/10.1016/s0967-0645(99)00037-5, 1999.
Measures, C. I. and Vink, S.: On the use of dissolved aluminum in surface waters to estimate dust deposition to the ocean, Global Biogeochem. Cy., 14, 317–327, https://doi.org/10.1029/1999gb001188, 2000.
Menzel Barraqueta, J.-L., Samanta, S., Achterberg, E. P., Bowie, A. R., Croot, P., Cloete, R., De Jongh, T., Gelado-Caballero, M. D., Klar, J. K., Middag, R., Loock, J. C., Remenyi, T. A., Wenzel, B., and Roychoudhury, A. N.: A first global oceanic compilation of observational dissolved aluminum data with regional statistical data treatment, Front. Mar. Sci., 7, 468, https://doi.org/10.3389/fmars.2020.00468, 2020.
Michalopoulos, P., Aller, R. C., and Reeder, R. J.: Conversion of diatoms to clays during early diagenesis in tropical, continental shelf muds, Geology, 28, 1095–1098, https://doi.org/10.1130/0091-7613(2000)28<1095:codtcd>2.0.co;2, 2000.
Moran, S. B. and Moore, R. M.: Evidence from mesocosm studies for biological removal of dissolved aluminum from sea-water, Nature, 335, 706–708, https://doi.org/10.1038/335706a0, 1988.
Nelson, D. M., DeMaster, D. J., Dunbar, R. B., and Smith, W. O.: Cycling of organic carbon and biogenic silica in the Southern Ocean: Estimates of water-column and sedimentary fluxes on the Ross Sea continental shelf, J. Geophys. Res.-Ocean., 101, 18519–18532, https://doi.org/10.1029/96jc01573, 1996.
Panagiotopoulos, C., Goutx, M., Suroy, M., and Moriceau, B.: Phosphorus limitation affects the molecular composition of Thalassiosira weissflogii leading to increased biogenic silica dissolution and high degradation rates of cellular carbohydrates, Org. Geochem., 148, 104068, https://doi.org/10.1016/j.orggeochem.2020.104068, 2020.
Ragueneau, O., Schultes, S., Bidle, K., Claquin, P., and Moriceau, B.: Si and C interactions in the world ocean: Importance of ecological processes and implications for the role of diatoms in the biological pump, Global Biogeochem. Cy., 20, GB4S02, https://doi.org/10.1029/2006GB002688, 2006.
Ren, H., Brunelle, B. G., Sigman, D. M., and Robinson, R. S.: Diagenetic aluminum uptake into diatom frustules and the preservation of diatom-bound organic nitrogen, Mar. Chem., 155, 92–101, https://doi.org/10.1016/j.marchem.2013.05.016, 2013.
Ren, J. L., Zhang, G.-L., Zhang, J., Shi, J.-H., Liu, S.-M., Li, F.-M., Jin, J., and Liu, C.-G.: Distribution of dissolved aluminum in the Southern Yellow Sea: Influences of a dust storm and the spring bloom, Mar. Chem., 125, 69–81, https://doi.org/10.1016/j.marchem.2011.02.004, 2011.
Riebesell, U.: Photosynthesis – Carbon fix for a diatom, Nature, 407, 959–960, https://doi.org/10.1038/35039665, 2000.
Schlitzer, R., Anderson, R. F., Dodas, E. M., Lohan, M., Geibert, W., Tagliabue, A., and Zurbrick, C.: The GEOTRACES Intermediate Data Product 2017, Chem. Geol., 493, 210–223, https://doi.org/10.1016/j.chemgeo.2018.05.040, 2018.
Shi, R., Li, G., Zhou, L., Liu, J., and Tan, Y.: The increasing aluminum content affects the growth, cellular chlorophyll a and oxidation stress of cyanobacteria Synechococcus sp. WH7803, Oceanol. Hydrobiol. Stud., 44, 343–351, https://doi.org/10.1515/OHS-2015-0033, 2015.
Smetacek, V.: Diatoms and the ocean carbon cycle, Protist, 150, 25–32, https://doi.org/10.1016/s1434-4610(99)70006-4, 1999.
Stallard, R. F.: Terrestrial sedimentation and the carbon cycle: Coupling weathering and erosion to carbon burial, Global Biogeochem. Cy., 12, 231–257, https://doi.org/10.1029/98gb00741, 1998.
Taylor, S. R.: Abundance of chemical elements in the continental crust – a new table, Geochim. Cosmochim. Ac., 28, 1273–1285, https://doi.org/10.1016/0016-7037(64)90129-2, 1964.
Tréguer, P. and Pondaven, P.: Silica control of carbon dioxide, Nature, 406, 358–359, https://doi.org/10.1038/35019236, 2000.
Tréguer, P., Bowler, C., Moriceau, B., Dutkiewicz, S., Gehlen, M., Aumont, O., Bittner, L., Dugdale, R., Finkel, Z., Iudicone, D., Jahn, O., Guidi, L., Lasbleiz, M., Leblanc, K., Levy, M., and Pondaven, P.: Influence of diatom diversity on the ocean biological carbon pump, Nat. Geosci., 11, 27–37, https://doi.org/10.1038/s41561-017-0028-x, 2017.
Twining, B. S., Baines, S. B., Vogt, S., and de Jonge, M. D.: Exploring ocean biogeochemistry by single-cell microprobe analysis of protist elemental composition, J. Eukaryot. Microbiol., 55, 151–162, https://doi.org/10.1111/j.1550-7408.2008.00320.x, 2008.
van Cappellen, P., Dixit, S., and van Beusekom, J.: Biogenic silica dissolution in the oceans: Reconciling experimental and field-based dissolution rates, Global Biogeochem. Cy., 16, 23-21–23-10, https://doi.org/10.1029/2001GB001431, 2002.
Van Bennekom, A. J., Buma, A. G. J., and Nolting R. F.: Dissolved aluminum in the Weddell-Scotia Confluence and effect of Al on the dissolution kinetics of biogenic silica, Mar. Chem., 35, 423–434, https://doi.org/10.1016/S0304-4203(09)90034-2, 1991.
van Hulten, M. M. P., Sterl, A., Middag, R., de Baar, H. J. W., Gehlen, M., Dutay, J.-C., and Tagliabue, A.: On the effects of circulation, sediment resuspension and biological incorporation by diatoms in an ocean model of aluminium, Biogeosciences, 11, 3757–3779, https://doi.org/10.5194/bg-11-3757-2014, 2014.
Wallmann, K., Aloisi, G., Haeckel, M., Tishchenko, P., Pavlova, G., Greinert, J., Kutterolf, S., and Eisenhauer, A.: Silicate weathering in anoxic marine sediments, Geochim. Cosmochim. Ac., 72, 2895–2918, https://doi.org/10.1016/j.gca.2008.03.026, 2008.
Xie, J., Bai, X., Lavoie, M., Lu, H., Fan, X., Pan, X., Fu, Z., and Qian, H.: Analysis of the proteome of the marine diatom Phaeodactylum tricornutum exposed to aluminum providing insights into aluminum toxicity mechanisms, Environ. Sci. Technol., 49, 11182–11190, https://doi.org/10.1021/acs.est.5b03272, 2015.
Yuan, P., Liu, D., Zhou, J. M., Tian, Q., Song, Y. R., Wei, H. H., Wang, S., Zhou, J. Y., Deng, L. L., and Du, P. X.: Identification of the occurrence of minor elements in the structure of diatomaceous opal using FIB and TEM-EDS, Am. Mineral., 104, 1323–1335, https://doi.org/10.2138/am-2019-6917, 2019.