the Creative Commons Attribution 4.0 License.
the Creative Commons Attribution 4.0 License.
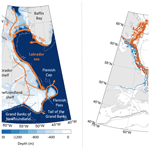
Circulation of Baffin Bay and Hudson Bay waters on the Labrador shelf and into the subpolar North Atlantic
Nicholas P. Foukal
Eleanor Frajka-Williams
In the coming decades increasing amounts of freshwater are predicted to enter the subpolar North Atlantic from Greenland and the Arctic. If this additional freshwater reaches the regions where deep convection occurs, it could potentially dampen ventilation and the formation of deep waters. In this study, we use a surface drifter dataset spanning the period 1990–2023 to investigate the pathways followed by waters originating from Davis Strait and Hudson Strait on the Labrador shelf and into the interior subpolar North Atlantic. Recent drifter deployments in the region allow for an improved understanding of the circulation on the Labrador shelf, in particular its northern part, where prior data were sparse. We show that waters originating from Davis Strait and Hudson Strait remain on the shelf as they flow downstream until they reach the Newfoundland shelf. This confirms that very little exchange takes place between the Labrador shelf and the interior Labrador Sea. Decomposing the Labrador shelf into five regions, we further describe typical pathways for these waters and show that extensive exchanges take place between the coastal and shelf-break branches of the Labrador Current. Our results suggest that if an increasing amount of freshwater reaches the Labrador shelf, it would not directly affect the Labrador Sea convection region; instead, it would lead to the formation of a salinity anomaly off the Grand Banks, which could then circulate around the subpolar North Atlantic.
- Article
(9336 KB) - Full-text XML
-
Supplement
(1345 KB) - BibTeX
- EndNote
In the interior seas of the subpolar North Atlantic, intense winter heat fluxes and relatively weak stratification allow for vertical mixing to depths reaching more than 1000 m (Lazier, 1980; Swift and Aagaard, 1981; de Jong et al., 2012), leading to the formation of dense waters. This process, known as deep convection, allows for the ventilation of the intermediate to deep ocean and contributes to the trapping of large amounts of anthropogenic carbon at depth (Steinfeldt et al., 2009; Rhein et al., 2017). A portion of the dense waters formed in these basins is then exported southwards as the lower limb of the Atlantic Meridional Overturning Circulation (Buckley and Marshall, 2016; Buckley et al., 2023; Lozier, 2023).
In the coming decades, climate change is predicted to cause an increase in the amount of freshwater entering the subpolar North Atlantic, from Greenland (Bamber et al., 2018; The IMBIE team, 2020) and the Arctic (Haine et al., 2015; Shu et al., 2018). This increase would supplement interannual variations in freshwater input to the region, for instance related to changes in the Beaufort Gyre circulation (Timmermans and Toole, 2023), which has recently been stabilizing after a long period of freshwater accumulation (Lin et al., 2023). Increased freshwater fluxes to the subpolar North Atlantic could lead to a freshening of the upper layers in areas where deep convection usually happens, which could increase the stratification and in turn weaken vertical mixing and deep water formation.
However, the freshwater that originates from Greenland and from the Arctic is first transported by narrow boundary currents over the continental shelves of Greenland and North America (Marsh et al., 2010). The freshwater must be mixed into the interior before it can affect the stratification of deep convection regions. Freshwater pathways from the shelf regions to the deep convection regions are complex, and there is no agreement on a number of issues related to this topic: the volume of freshwater that would need to enter these regions to affect deep convection, whether Greenland freshwater is already affecting salinity and deep convection, and how far in the future this could occur (Böning et al., 2016; Yang et al., 2016; Dukhovskoy et al., 2019; Schiller-Weiss et al., 2024).
In the Labrador Sea, most exchanges between the shelf and interior have been identified at the West Greenland shelf, near Cape Desolation. There, winds and eddies drive freshwater originating from Fram Strait and East Greenland glaciers into the central Labrador Sea (Lilly et al., 2003; Luo et al., 2016; Schulze Chretien and Frajka-Williams, 2018). In contrast, on the Labrador shelf, little to no export of shelf waters into the interior is thought to take place (Myers, 2005; Pennelly et al., 2019), with only very limited cross-shelf exchanges described by observational studies (Howatt et al., 2018; Clément et al., 2023). While the waters exported near Cape Desolation mostly originate from Fram Strait and East Greenland glaciers (Castelao et al., 2019), waters found on the Labrador shelf also originate from West Greenland glaciers (Gillard et al., 2016), the Arctic via Davis Strait (Lazier and Wright, 1993), and Hudson Bay (Straneo and Saucier, 2008). To understand the possible impact of increased freshwater fluxes from Greenland and the Arctic on the subpolar North Atlantic, it is therefore essential to understand how the waters that enter the Labrador shelf circulate over the shelf and where and how they leave it to enter the open ocean.
Our understanding of the circulation on the Labrador shelf dates back from early oceanographic expeditions of the late 19th and early to mid-20th century (Smith et al., 1937) and was informed by repeated summer sections along the shelf, as well as mooring arrays from the late 20th century onwards (Lazier and Wright, 1993; Cyr and Galbraith, 2021). At the northern end of the Labrador shelf, the Baffin Island Current transporting Baffin Bay and West Greenland Current waters (Cuny et al., 2005; Curry et al., 2014), the Hudson Strait outflow (Straneo and Saucier, 2008; Ridenour et al., 2021), and the remaining waters from the West Greenland Current come together to form the Labrador Current (Fig. 1a). The Labrador Current is composed of three branches (Lazier and Wright, 1993; Fratantoni and Pickart, 2007): (1) an outer, barotropic branch, found around the 1500 m isobath; (2) a main, surface intensified branch, centred at the 500 m isobath; and (3) an inner branch that closely follows the coast, also referred to as the Labrador Coastal Current. In the following we refer to the three branches as “slope”, “shelf-break”, and “coastal” Labrador Current.
The shelf-break Labrador Current is typically indistinguishable from the slope Labrador Current in velocity sections, but the water masses it transports are colder and fresher (Lazier and Wright, 1993; Fratantoni and Pickart, 2007). While the coastal Labrador Current can be distinguished as a separate branch, its origins are disputed, with the literature both suggesting it stems from the Hudson Strait outflow (Florindo-López et al., 2020) or from the steering of waters into the deep troughs and canyons that cut across the shelf (Peterson, 1987; Colbourne et al., 1997; Fig. 1b). The salinity of waters transported in the Labrador Current is driven by seasonal variations in river discharge and ice melt. Peak freshness of surface waters is observed at the Labrador shelf in early summer, and it reaches the Newfoundland shelf in late summer (Fratantoni and McCartney, 2010).
As it reaches Newfoundland, the coastal current bifurcates towards the shelf edge to join the shelf-break current (Colbourne et al., 1997; Fratantoni and McCartney, 2010). A limited part of the coastal current remains close to the coast and flows through the Strait of Belle Isle, north of Newfoundland, or towards the Avalon Channel, east of Newfoundland (Petrie and Anderson, 1983; Shaw and Galbraith, 2023). At the shelf edge, the Labrador Current splits as it reaches Flemish Cap: one branch enters Flemish Pass, while another rounds Flemish Cap. Between Flemish Cap and the Tail of the Grand Banks, the Labrador Current is retroflected towards the subpolar North Atlantic and loses approximately 90 % of its volume transport (Lazier and Wright, 1993; Loder et al., 1998; Fratantoni and Pickart, 2007). The strength of the retroflection, and therefore the amount of waters diverted from the Labrador shelf into the subpolar North Atlantic, varies on seasonal and interannual timescales, modulated by the large-scale wind patterns and the strength of the Labrador Current (Petrie and Drinkwater, 1993; Jutras et al., 2023; Gonçalves Neto et al., 2023; Furey et al., 2023).
The goal of this study is to characterize the pathways followed by freshwater originating from Baffin Bay and Hudson Bay on the Labrador shelf and into the subpolar North Atlantic, as well as to better understand the possible downstream impact of increased freshwater fluxes from these regions. We investigate these pathways using surface drifters. In Sect. 3.1, we describe the surface circulation as inferred from the drifter dataset and compare it with existing studies. In Sect. 3.2, we investigate exchanges between the inner Labrador shelf, where the fresh waters are found, and the central Labrador Sea, where deep convection takes place. In Sect. 3.3, we define the typical pathways of drifters originating in Baffin Bay and Hudson Bay over the Labrador shelf. In Sect. 3.4, we investigate where these drifters enter the interior ocean and how they spread across the subpolar North Atlantic. In Sect. 4, we put our results in the context of existing studies and discuss what these freshwater pathways imply for the future influence of waters originating from Baffin Bay and Hudson Bay on deep convection in the subpolar North Atlantic.
2.1 Surface drifters
We use surface drifter trajectories from the Global Drifter Program (GDP) 6 h interpolated dataset (Centurioni et al., 2019; Lumpkin and Centurioni, 2019) and from the East Greenland Current Drifter Investigation of Freshwater Transport dataset (EGC-DrIFT; Duyck and De Jong, 2023b). From the GDP, we use all available data from January 1990 to May 2023.
From EGC-DrIFT, we use Surface Velocity Program (SVP) drifters that are similarly interpolated every 6 h between August 2019 and November 2022 (Duyck and De Jong, 2023a). The SVP drifters from both datasets are fitted with a holey sock drogue, centred at 15 m depth, which ensures limited wind drift and allows them to follow the circulation at that depth.
We filter the drifter trajectories with a 25 h Butterworth filter to remove the high-frequency signal due to tides and inertial oscillations and recompute drifter velocities from the filtered trajectories using a central difference algorithm. To ensure the quality of the dataset, we exclude drifters that have lost their drogues and cut the trajectories of drifters that grounded ashore or did not transmit for more than 30 d in a row. The result of this filtering is shown in Fig. S1 in the Supplement.
Since 2019, several drifter deployments took place at the Greenland shelf, motivated by the growing interest in the interactions between fresh continental shelves and the deep convection regions. While a few studies already investigated surface circulation at the Labrador shelf using drifters (Reverdin et al., 2003; Cuny et al., 2002; Fratantoni, 2001), they were limited regionally due to the paucity of drifter trajectories on the whole shelf. Even with the new data collected since 2019, the drifter coverage of the region is still heterogeneous, with an order of magnitude more drifter data available over the central Labrador Sea compared to the Labrador shelf (Fig. 2a). However, the large increase in available data over the whole region also included the northern Labrador shelf, where little to no drifter data were previously available (Fig. 2b).
While these new data allow for new analysis of the circulation on the Labrador shelf, the resulting time concentration of the dataset can lead to bias: out of 35 drifters that passed through Davis Strait, 25 were deployed at the same time, in September 2021, as part of the TERIFIC (Targeted Experiment to Reconcile Increased Freshwater with Increased Convection) experiment. Moreover, sea ice cover prevents drifters from flowing over most of the Labrador shelf in winter and spring, and it may also affect their behaviour in the late autumn: part of the TERIFIC drifters mentioned above were caught in sea ice as they flowed over the Labrador shelf, resulting in a lower amount of available data in that region in late winter and early spring (See Fig. S2 for drifter trajectories superimposed on sea ice maps). There are moreover inherent limitations to the drifter dataset: while the SVP drifters allow inferences of the ocean circulation at 15 m depth and can give an indication of freshwater pathways, they do not directly track freshwater. Despite these limitations, the drifter dataset can provide useful additional results in a poorly sampled area and provide a complementary perspective to the existing literature.
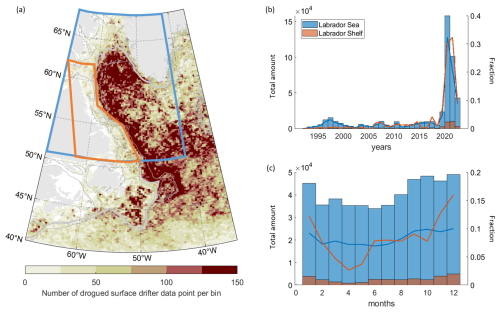
Figure 2(a) Number of drifter data points per bin from 1990 to 2023. Bins are ° latitude by ° longitude. Bathymetry is shown in grey: 2500, 1000, and 250 m isobaths, with the smoothed 1000 m isobath used as the indication of the shelf break in bold. The red and blue boxes show the regions used for the histograms, defined as the Labrador Sea and Labrador shelf regions. (b) Number of drifter data points per year in the Labrador Sea area (blue) and Labrador shelf area (red) as defined in (a). The bar plot shows the total number of points per year in the region, and the line plot shows the fraction of these points that is available in a given year. (c) Same as (a), but for each month.
2.2 Definition of the shelf boundary and drifter crossing computation
To identify cross-shelf exchanges in the Labrador Sea, we define a shelf boundary using the 1000 m isobath. The choice of this isobath is motivated by the need for a boundary that works well for both the West Greenland and Labrador shelf, the former having a steeper slope than the latter. We smooth the shelf boundary with a 150 km window and interpolate it to a resolution of 5 km. The resulting shelf boundary is shown in Fig. 3a, together with the along-shelf distance from Cape Farewell. To detect where and when drifters cross into or out of the shelf region, we divide the shelf boundary in 200 km segments and compute the number of crossings across each segment. Some drifters cross the shelf boundary multiple times. To identify locations of net offshore crossings we use the following criteria: if the drifters were on the shelf prior to crossing the segment of the shelf boundary, crossed it in the offshore direction more often than in the inshore direction, and did not cross back inshore at the five following segments (which corresponds to 1000 km), they are considered exported at that segment. These criteria also allow us to avoid the complex shape of the shelf boundary from affecting the results.
2.3 Drifter pathways over the Labrador shelf
Of the 35 drifters that entered the Baffin Island shelf via Davis Strait, only 5 reached the Newfoundland shelf while their drogues were still attached. The rest lost their drogues as they flowed over the Labrador shelf, were stranded at the coast, or were caught in sea ice. Instead of only considering drifters that flowed all the way from Baffin Bay and Hudson Bay to the Newfoundland region, we investigate the behaviour of drifters over smaller subsets of the Labrador shelf. To do so, we define five sections upstream of the Labrador shelf and as cross-shelf sections of the shelf, which are further divided into 11 boxes to differentiate between drifters flowing in the different branches of the Labrador Current.
The sections and boxes (shown Fig. 3b) are referred to as (1) the “origin” section, made up of the Davis Strait and West Greenland Current boxes, to differentiate the behaviour of drifters flowing from Baffin Bay and from the West Greenland Current; (2) the “northern Labrador shelf” section, at the mouth of Hudson Strait, with an inshore and offshore box to separate the Baffin Island Current and the remnant of the West Greenland Current; (3) the “mid-Labrador shelf” section at 56° N, with an inshore and offshore box to detect drifters steered towards the coast along the canyons that cut across the Labrador shelf; (4) the “southern Labrador shelf” section, just south of Hamilton bank, with an inshore and offshore box to differentiate the shelf-break and coastal current; and (5) the “Newfoundland” section, with an inshore box to identify drifters flowing over the shallow Newfoundland shelf and the Strait of Belle Isle, a Flemish Pass box, and a Flemish Cap box. The offshore end of each section extends to 100 km off the 1000 m isobath.
To resolve the pathways of drifters flowing over the Labrador Shelf, we examine their behaviour between pairs of sections. For each of these, we identify drifters found at both sections and classify them according to the boxes they originated from and arrived to. Sometimes, drifters passed through several boxes of the same section. If that is the first section of the pair, we consider the last box they left when leaving that section. If that is the second section of the pair, we consider the first box they entered when arriving at that section. From this, we can build an overview of the number of drifters flowing from one box to the next and preferred pathways, as well as the median travel time.
This section-to-section analysis increases the number of drifters we can include in our study, which limits time bias and enables the drawing of more robust conclusions. Instead of only 5 drifters, we use data from 218 different drifters that flowed across two or more of the defined sections. The additional drifters were deployed downstream of Davis Strait, recirculated onto the Baffin Island shelf or Labrador shelf after flowing with the West Greenland Current, and/or stopped working before they reached Newfoundland.
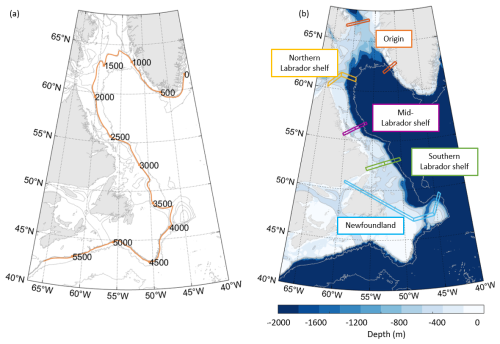
Figure 3(a) Definition of the shelf break as the smoothed 1000 m isobath and along-shelf distance from Cape Farewell in kilometres. Bathymetry is shown in grey: 5000, 2500, 1000, 500, and 250 m isobaths. (b) The 5 sections and 11 boxes are defined over the Labrador shelf to identify typical pathways for waters flowing from Davis Strait and Hudson Strait to the Newfoundland region. The boxes and legends are coloured according to the section they correspond to.
3.1 Surface circulation over the Labrador and Newfoundland shelves
In this section we build a map of the surface circulation from the drifter datasets to identify the main characteristics of surface currents in the Labrador Sea and compare the drifter-inferred circulation with existing knowledge, which was developed mostly from synoptic sections, moorings, and models. Using the full drifter dataset, from 1993 to 2023, we construct a pseudo-Eulerian map of the surface circulation in the western subpolar North Atlantic (Fig. 4). The map is built as ° latitude by ° longitude bins, with the velocity at each bin computed as the average velocity of all drifter data available in the region corresponding to that bin. Bins are only shown if they represent more than five data points from at least two separate drifters.
The main export of shelf waters into the Labrador Sea takes place at the west Greenland shelf between Cape Desolation (60° N) and Fylla Bank (63° N). This is visible in Fig. 4 as a broadening and dissipating of part of the West Greenland Current into the Labrador Sea. Past Fylla Bank, three distinct branches of the West Greenland Current are distinguishable: (1) part of the West Greenland Current is steered westwards, between the 1000 to 2500 m isobaths; (2) another part continues northwards along the 500 m isobath and recirculates into the Baffin Island Current as it reaches Davis Strait; and (3) a small portion of the flow continues northwards along the west Greenland shelf into Baffin Bay.
The Baffin Island Current is composed of waters both originating from Baffin Bay and recirculating from the West Greenland Current. In addition to the main branch of the Baffin Island Current, situated at the 500 m isobath, the drifter dataset shows a secondary flow close to the Baffin Island coast, which could be a coastal branch of the Baffin Island Current. However, the drifters which contributed to the pseudo-Eulerian map in this coastal flow were all deployed in September 2021. We therefore cannot conclude whether it is a permanent feature only from using these data (Fig. S2a shows more clearly the drifters that circulate in the main branch of the Baffin Island Current and closer to the coast).
As it approaches Hudson Strait, the Baffin Island Current is steered towards its entrance. Part of the drifters found in the Baffin Island Current entered the Hudson Strait for a short time before recirculating back onto the shelf. Others that flowed inshore of the main branch of the Baffin Island Current entered Hudson Strait by its northernmost end and continued upstream into the strait. This is consistent with Ridenour et al. (2021) and with the drifters analysed in LeBlond et al. (1981, not included in this dataset), both of which show that the Hudson Strait inflow is of Baffin Bay origin.
Past Hudson Strait, the Hudson Strait outflow, the Baffin Island Current, and the remainder from the West Greenland Current combine at the Labrador shelf break to form the Labrador Current. Only one current core is visible at the shelf break, in agreement with the description of the Labrador Current from Lazier and Wright (1993). The available drifter data do not allow detection of a coastal branch of the Labrador Current north of 58° N. There is no clear connection between the Hudson Strait outflow and the coastal Labrador Current directly past Hudson Strait: of the 11 drifters that passed through Hudson Strait, only 2 drifters remained close to the coast as they entered the Labrador shelf. One of them beached shortly afterwards, while the other lost its drogue after being trapped in sea ice. The other nine drifters all joined the main branch of the Labrador Current at the shelf break (Fig. S2b).
Over the Labrador shelf, deep canyons drive exchanges between the shelf edge and the inner shelf. The Labrador shelf circulation map (Fig. 4b) shows three such features, at 58, 56, and 55° N, between Saglek Bank and Nain Bank, at the Hopedale Saddle, and at the Cartwright Saddle (See Fig. 1b for names of topographic features). In all cases, some of the drifters steered towards the coast were entrained in the coastal branch of the Labrador Current, while the remainder were steered back towards the shelf-break branch. This is very similar to the circulation that Peterson (1987) inferred from ice floes. The coastal branch of the Labrador Current is not visible along the whole Labrador shelf in the pseudo-Eulerian map, which is likely a consequence of the sparse drifter coverage over the inner shelf.
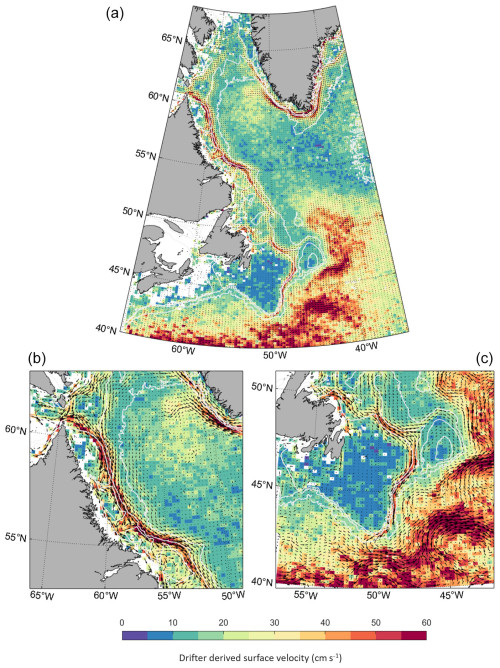
Figure 4(a) Pseudo-Eulerian map of the surface circulation in the western subpolar North Atlantic. Each bin shows the average drifter speed in a ° latitude by ° longitude region. Bins are shown only if they represent more than five data points and if these data points are from at least two different drifters. The bathymetry in white shows the 2500, 1000, and 250 m isobaths. (b) Same as (a), but for the Northern Labrador shelf. (c) Same as (a), but for the Newfoundland shelf.
Past Hamilton Bank, from 53° N, several pathways are visible from the shelf break over the shelf and into the coastal current through Hawke Saddle and Funk Island Deep. This enhanced exchange is consistent with previous work that showed drifters steered towards the coast in that region (Colbourne et al., 1997, not included in this dataset) and identified an increase in West Greenland Current origin waters over the shelf past Hamilton Bank (Benetti et al., 2017).
The circulation map shows three possible pathways for waters found in the coastal current as it reaches the Newfoundland shelf, though only the main one is clearly visible: (1) a few of the drifters are steered into the Strait of Belle Isle or (2) continue along the Newfoundland coast into the Avalon Channel (Petrie and Anderson, 1983), while (3) the majority of the drifters are channelled through the Bonavista Corridor towards the shelf-break Labrador Current.
As it reaches Flemish Cap, the Labrador Current splits. Part of the current is driven away from the shelf break and flows around Flemish Cap. The rest remains at the shelf break and flows through Flemish Pass. Between Flemish Pass and the Grand Banks, drifters are diverted away from the shelf break and entrained into the North Atlantic Current (Fig. 4c). This is clearest at the Tail of the Grand Banks. Past the Tail of the Grand Banks, no fast current is distinguishable at the shelf break anymore, consistent with the retroflection of the Labrador Current identified in that region (Fratantoni and Pickart, 2007; Jutras et al., 2023).
3.2 Cross-shelf exchanges into the central Labrador Sea
In this section, we investigate cross-shelf exchanges between the continental shelves and central Labrador Sea, as well as the origin of drifters that enter the interior Labrador Sea. We determine the regions where most exchanges occur by dividing the shelf boundary into 200 km along-shelf segments and identifying drifters that were exported from the shelf into the interior across these segments, as defined in Sect. 2.2 (Fig. 5).
There are two main regions where a substantial fraction of the drifters found on the continental shelves of the Labrador Sea are exported (Fig. 5a–b): (1) the eddy-shedding region of the west Greenland shelf and (2) Flemish Pass and the Grand Banks. Past the Grand Banks, the number of drifters found over the shelf strongly diminishes due to the retroflection of the Labrador Current. A minority of drifters also leave the shelf in the region just before Flemish Pass. In the following, we focus on exchanges taking place between the Labrador and Newfoundland shelves and the open ocean.
Example trajectories for drifters exported across groups of segments of the shelf boundary show that not only different areas of the shelf have less or more exchanges, but also it is partly drifters of different origins that are exported in different areas: the drifters that crossed the shelf boundary from the Labrador shelf into the central Labrador Sea before reaching the Newfoundland shelf nearly only originated from the West Greenland Current (Fig. 5c–d). By contrast, the drifters exported past Flemish Pass originated from both the West Greenland Current and from Baffin Bay and Hudson Bay, with part of these drifters circulating over the inner shelf before they reached Flemish Pass (Fig. 5e).
These results suggest a distinction in the origin and fate of waters transported by different branches of the Labrador Current: waters transported in the slope Labrador Current, which originate from the West Greenland Current, remain at the offshore edge of the shelf and can be exported into the central Labrador Sea. These are however not particularly fresh waters. Waters transported by the shelf-break and coastal Labrador Current, which notably includes very fresh waters of Hudson Strait and Davis Strait origin, only leave the shelf further downstream, around Flemish Cap and the Grand Banks.
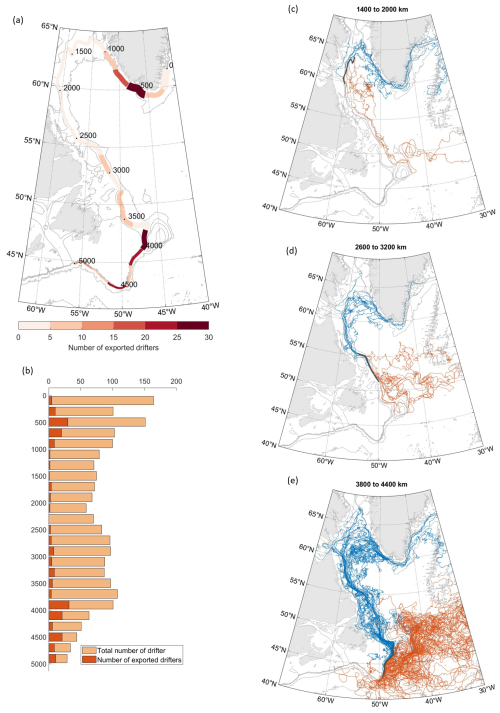
Figure 5(a) Number of drifters exiting the shelf at a given segment of the shelf boundary. The width of the segment is proportional to the total number of drifters found over the shelf at that segment (within 100 km of the shelf edge, also shown in b). Drifters are not considered if they immediately re-enter the shelf at the next segment. (b) Total number of drifters (pale orange) and number of drifters leaving the shelf (red) at a given segment of the shelf boundary. (c, d, e) Three examples of drifter trajectories before (blue) and after (red) they leave the shelf at a given segment of the shelf (composed of three of the 200 km segments, shown in black). For all panels, bathymetry is shown in grey: 5000, 2500, 1000, 500, and 250 m isobaths.
To further this analysis, we look specifically at how drifters originating from Davis Strait and Hudson Strait flow over the Labrador shelf and enter the subpolar North Atlantic (Fig. 6). Drifters from these two origins, shown respectively in red and in blue, display a similar behaviour. The large majority of the drifters remained over the shelf until they reached the Newfoundland shelf and were only exported into the subpolar North Atlantic at, and past, Flemish Pass. Only one of the drifters crossed the shelf boundary into the Labrador Sea (blue trajectory, between 56 and 52° N), and it remained in the Labrador Current downstream. This could be a clue for local exchanges between the shelf and interior. We also remark that some of the Hudson Strait drifters spread over the inner Labrador shelf earlier than Davis Strait drifters, though it does not seem to lead to differences in their downstream trajectories.
The analysis of both cross-shelf exchanges along the Labrador shelf and the trajectories of drifters originating from Davis Strait and Hudson Strait suggest little direct connection between Baffin Bay and Hudson Bay and the interior Labrador Sea. These drifters are only exported away from the shelf downstream of Flemish Pass. In addition, the spreading of drifters over the shelf as they flow downstream suggests extensive exchanges between the shelf-break branch of the Labrador Current and its coastal branch.
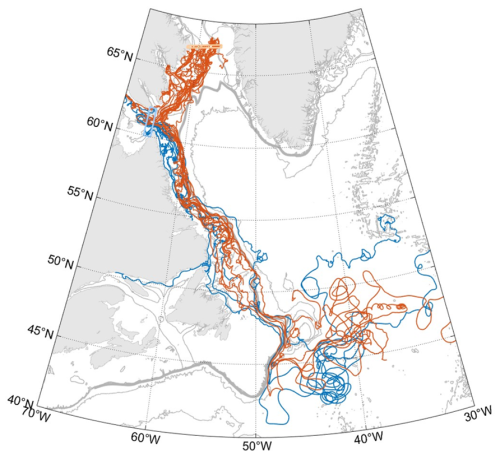
Figure 6Trajectories of drifters originating from or passing through Davis (red) and Hudson (blue) straits. Drifters that originate from Davis Strait but also enter and exit Hudson Strait are marked as Hudson Strait drifters. The boxes used to identify drifters crossing the straits are shown in light red and light blue. Bathymetry is shown in grey: 5000, 2500, 1000, 500, and 250 m isobaths.
3.3 Pathways of Baffin Bay and Hudson Bay waters over the Labrador shelf
Only a few of the drifters found at Davis Strait and/or Hudson Strait were also found at the Newfoundland shelf. In the following we apply the method described in Sect. 2.3 to characterize drifter pathways over the Labrador shelf, which allows us to also use drifters that flowed over only part of the shelf.
Between the Davis Strait box and northern Labrador shelf section, drifters mostly remain over the Baffin Island shelf: nearly all (11 out of 12; Fig. 7) the drifters originating from the Davis Strait box cross the inshore box of the northern Labrador shelf section (Fig. 8a). These drifters are joined at that inshore box by some of the drifters that recirculated from the West Greenland Current into the Baffin Island Current, as well as drifters from the Hudson Strait outflow. By contrast, drifters originating from the West Greenland box tend to remain over the slope, and more than 90 % of these flowed through the offshore box at the northern Labrador shelf section.
Between the inshore box of the northern Labrador shelf section and the southern Labrador shelf section, the drifters largely remain on the shelf but display strong exchanges between the shelf-break and coastal branches of the Labrador Current (Fig. 8b). Drifters originating from the inshore box of the northern Labrador shelf section first join the Labrador Current at the shelf break. Most are then steered towards the coast via the canyons that cut across the Labrador shelf: at the mid-Labrador shelf section, 75 % of these drifters are found in the inshore box, which corresponds to the coastal Labrador Current and to the circulation along the topography of the canyons. While 64 % of the drifters found in the inshore box at the mid-Labrador shelf section remain in the coastal current downstream until the southern Labrador shelf section, the other 36 % are driven back towards the shelf edge and found in the shelf-break Labrador Current by the southern Labrador shelf section (Fig. 7).
By contrast, between the offshore box of the northern Labrador shelf section and the mid-Labrador shelf section, drifters remain at and off the shelf break. Limited exchanges with the inner shelf only take place between the mid- and southern Labrador shelf sections, past Hamilton Bank (Fig. 8d). The large majority (94 %) of the drifters found in the offshore box at the northern Labrador shelf section are also found in the offshore box at the mid-Labrador shelf section. Between the mid- and southern Labrador shelf sections, 15 % of the drifters originally found at the shelf break enter the inner shelf and are found in the inshore box of the southern Labrador shelf section (Fig. 7). This behaviour is shown by the drifter trajectories drawn in purple in Fig. 8c that remain at and off the shelf break upstream of Hamilton Bank and only spread over the shelf from and downstream of Hamilton Bank. The one drifter from Davis Strait that crosses the offshore box at the northern Labrador shelf section follows this latter pathway.
In summary, until 54° N, the drifters found at the offshore box at the northern Labrador shelf section mostly remain at the shelf break and off the shelf, while drifters found at the inner box spread over the inshore shelf. Downstream of 54° N, drifters that were found at the inshore box continue showing exchanges between the coastal and shelf-break branch, but part of the drifters that originated from the offshore box also take part in these exchanges.
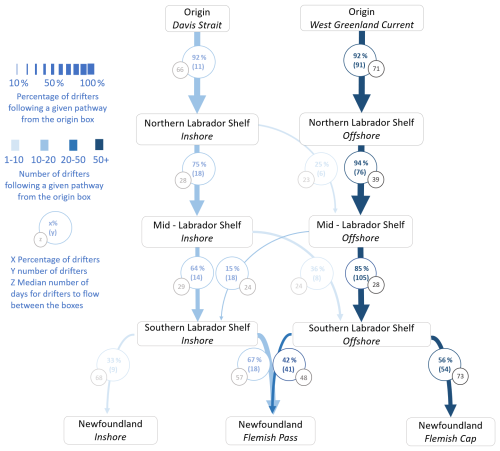
Figure 7Pathways followed by drifters from Davis Strait and the West Greenland shelf to the Newfoundland shelf and the Grand Banks. All computations correspond to drifters flowing from a given origin box of a first section to a second section and therefore only consider drifters present at both sections. The width of the arrows indicates the percentage of drifters following a given path from the origin box, while the intensity of the colour indicates the number of drifters following that path. This information is also shown in the circles drawn over the arrows. The grey circles show the median number of days drifters took to go from one box to the next. Only paths that are followed by more than 10 % of drifters from a given box are shown.
Between the inshore box of the southern Labrador shelf section, which corresponds to the coastal Labrador Current, and the Newfoundland section, three distinct behaviours can be observed (Fig. 8d). The drifters that remain at the inner shelf and cross the inshore box at the Newfoundland section (33 % of the drifters found in the coastal current at the southern Labrador shelf section) either enter the Strait of Belle Isle or flow along the Newfoundland coast towards the Avalon Channel. The remaining 67 % flow towards the shelf break and are found at the Flemish Pass box when they reach the Newfoundland section. None of the drifters originating from the inshore box of the southern Labrador shelf section were found at the Flemish Cap box at the Newfoundland section. This confirms that in our dataset, drifters found in the coastal current over the southern Labrador shelf only exit the shelf downstream of Flemish Pass.
Between the offshore box of the southern Labrador shelf, which corresponds to the shelf-break and slope Labrador Current, and the Newfoundland section, the large majority of drifters either flowed through Flemish Pass (42 %) or circulated around Flemish Cap (56 %). Only two drifters crossed the inshore box, flowing over the Newfoundland shelf slightly west of Flemish Pass. Figure 8f suggests that the drifters that circulated around Flemish Cap remained offshore of the Labrador shelf before reaching that region (trajectories in green), while the drifters that at some point circulated over the shelf were either driven into the Flemish Pass (in blue) or flowed over the inshore Newfoundland shelf (in red).
This is confirmed when also investigating trajectories directly between the northern Labrador shelf and Newfoundland sections (Figs. S4 and S5): only 13 of the drifters found at the inshore box of the northern Labrador shelf section were still active when they reached the Newfoundland section. Of these, 11 crossed the Flemish Pass box, with the 2 others crossing the inshore box after flowing through the Strait of Belle Isle and along the Newfoundland coast. On the other hand, the 46 drifters that originated from the offshore box of the northern Labrador shelf section and were still active at the Newfoundland section are distributed equally between the Flemish Cap and Flemish Pass boxes, with 46 % of these drifters flowing through each box.
The drifter pathways suggest that waters from Hudson and Davis Strait, found at the inner shelf upstream of the Labrador shelf, remain over the Labrador shelf as they flow downstream, only entering the open ocean downstream of Flemish Pass. By contrast, the drifters suggest that waters originating from the West Greenland Current and found at the shelf break and off the shelf remain offshore while flowing along most of the Labrador shelf, partly entering the shelf at Hamilton Bank. When adding the times taken by drifters to flow between the different sections, we find that they take a median time of 180 d from Davis Strait to Flemish Pass if they flow in the coastal branch of the Labrador Current over the shelf and 165 d if they flow in the shelf-break current (Fig. 7).
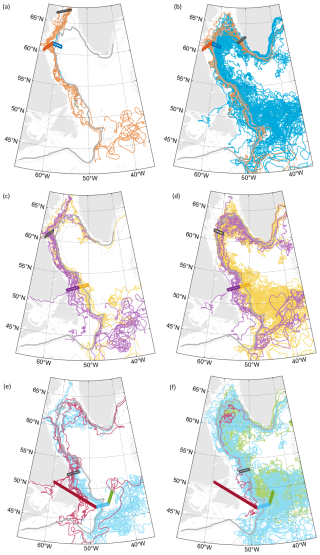
Figure 8Trajectories of drifters between (a, b) the origin and northern Labrador shelf sections, (c, d) the northern and southern Labrador shelf sections, and (e, f) the southern Labrador shelf and Newfoundland sections. The black box shows the origin box of the drifters displayed, while the coloured boxes show the different boxes of the end section. Drifters are coloured according to which box of the end section they cross first. The bathymetry is shown in grey with the 5000, 2500, 1000, 500, and 250 m isobaths, and the shelf boundary is shown as a thick grey line.
3.4 Export of surface drifters at Flemish Cap and the Grand Banks
In this section, we investigate where drifters are exported from the shelf after passing Flemish Pass and Flemish Cap and how they then circulate around the subpolar North Atlantic.
The drifters that flowed through Flemish Pass exit the Labrador Current between Flemish Pass and past the Tail of the Grand Banks. To investigate more exactly where they leave the shelf and are entrained into the interior subpolar North Atlantic, we use the along-shelf distance from Cape Farewell (as defined Sect. 2.2) to identify where drifters stop following the shelf boundary and retroflect. We use three along-shelf distances (Fig. 9): 4000 km, at the southern end of Flemish Pass; 4300 km, upstream of the Tail of the Grand Banks; and 4600 km, downstream of the Tail of the Grand Banks.
The drifters that stopped following the shelf before and at the southern end of Flemish Pass (19 of the 105 drifters that crossed Flemish Pass) were entrained in the anticyclonic circulation around Flemish Cap or bifurcated northeastwards at the sharp bathymetric bend south of Flemish Pass (Fig. 9a). These drifters entered the interior ocean south of Flemish Cap.
Drifters that reached the Grand Banks and stopped following the shelf before the Tail of the Grand banks bifurcated northeastwards over that whole area (Fig. 9b), in a similar way as the drifters exported at the Tail of the Grand Banks (Fig. 9c). This shows that the retroflection of the Labrador Current takes place from the southern end of Flemish Pass to the Tail of the Grand Banks, even as it seems to be intensified in that latter region (29 drifters stopped following the shelf break between Flemish Pass and the Tail of the Grand Banks, 44 at the Tail of the Grand Banks). This is consistent with previous studies of the retroflection, such as Fratantoni and Pickart (2007) and Gonçalves Neto et al. (2023), who also showed that the retroflection area can additionally vary over time.
In our dataset, all the drifters that were still found in the Labrador Current past the Tail of the Grand Banks had left the shelf by the Laurentian Channel. After crossing the shelf boundary, these drifters flowed eastwards and later northeastwards, following a similar trajectory to the drifters retroflected at the Grand Banks (Fig. 9d). This is in contrast to earlier work; for instance Jutras et al. (2023) showed that 25 % of the particles transported in the Labrador Current continued downstream over the Scotian shelf and Gulf of Maine between 1993 and 2015. This difference could be due to a temporal bias in the drifter dataset toward more recent years, as well as a heterogeneous seasonal coverage, with 4 times more drifters found over the Newfoundland shelf in August than February (Gonçalves Neto et al., 2023).
The drifters that left the shelf before the Flemish Pass box and circulated around Flemish Cap also exhibit a range of behaviours, illustrated in Fig. S6. There are two main areas where drifters left the circulation around Flemish Cap: in the northern part of Flemish Cap only drifters that were found past the 2500 m isobath at the Flemish Cap box were exported. Most drifters were exported in the southeast and southern part of Flemish Cap, where the bathymetry is the steepest. This is notably the case of the drifters that rounded Flemish Cap above its shallowest parts. The drifters leave the circulation around Flemish Cap in the same regions (northeast and south of Flemish Cap) that Solodoch et al. (2020) described for the Deep Western Boundary Current.
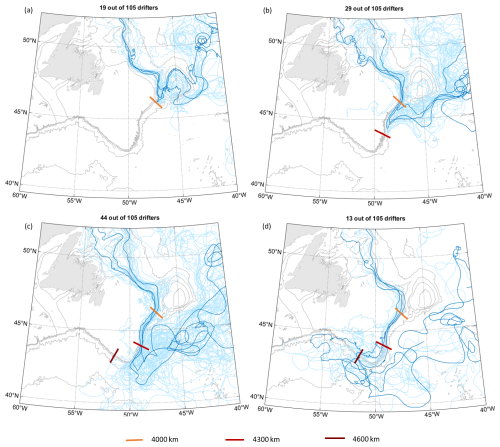
Figure 9Behaviour of drifters after they flow through the Flemish Pass box. The panels show drifters detrained from the Labrador Current (a) before reaching the southern end of Flemish Pass (4000 km along-shelf from Cape Farewell), (b) between the southern end of Flemish Pass and the Tail of the Grand Banks (4000–4300 km), (c) at the Tail of the Grand Banks (4300–4600 km), and (d) past the Tail of the Grand Banks (beyond 4600 km along-shelf from Cape Farewell). In all cases, the darker-blue trajectories represent examples of trajectories for the group of drifters. The bathymetry is shown in grey with the 5000, 2500, 1000, 500, and 250 m isobaths.
The upstream origin of the drifters influences where they are exported in the Newfoundland–Flemish Cap region. While a subset of the drifters found in the slope and shelf-break Labrador Current round Flemish Cap seaward and are entrained into the North Atlantic Current northeast of Flemish Cap, drifters originating from the coastal current do not leave the shelf break before they reach the southern end of Flemish Pass and the Grand Banks (Fig. 10). This is also the case more generally for drifters coming from Davis Strait and Hudson Strait, as shown in the previous section. However, even as there is initially a strong difference in where the drifters are detrained from the Labrador Current, this difference disappears as they are entrained in the North Atlantic Current and start recirculating into the subpolar gyre. Most of these drifters then remain in the subpolar North Atlantic, with a few leaving the North Atlantic Current to the south, possibly heading into the subtropical gyre.
We find that it takes a median time of 196 d, nearly 7 months, for drifters to reach 30° W from the Flemish Pass section (204 d from Flemish Cap). In the previous section, we showed that it takes a median time of 180 d for the drifters included in our dataset to flow from Davis Strait to Flemish Pass. According to this dataset, it would therefore take more than a year for an anomaly to spread from Davis Strait to the mid-Atlantic ridge.
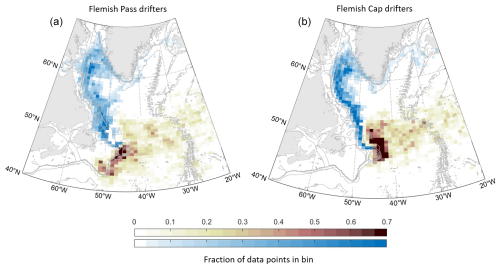
Figure 10Distribution of drifter data from the drifters that passed through the Flemish Pass (a) and Flemish Cap (b) boxes. For both Flemish Cap and Flemish Pass drifters, the coloured bins show the fraction of the total number of data points from these drifters found in a given bin, with in blue the data collected before the drifters crossed the box and in brown after the drifter crossed the box. Drifter data are only considered 1 year before and after the drifter crossed the box. The bathymetry is shown in grey with the 5000, 2500, 1000, 500, and 250 m isobaths.
4.1 Circulation of Baffin Bay and Hudson Bay waters over the Labrador shelf and into the subpolar North Atlantic
Our results suggest that waters found at the inner shelf near Hudson Strait – which includes Baffin Bay waters, Hudson Bay waters, and part of the waters recirculating from the West Greenland Current – remain on the shelf until Flemish Pass as part of the coastal and shelf-break branches of the Labrador Current. By contrast, waters originating from the West Greenland Current and found on the outer shelf near Hudson Strait seem to remain in the slope branch of the Labrador Current until past Hamilton Bank, where some are steered further onto the shelf and into the coastal current. Most of the waters circulating over the inner Labrador shelf are exported to the subpolar North Atlantic between Flemish Pass and just past the Tail of the Grand Banks. There, they enter the North Atlantic Current and follow its path downstream. In the following, we discuss exchanges between the shelf and the interior regions, between the coastal and shelf-break branches of the Labrador Current, and between the shelf-break and slope branches of the Labrador Current.
The drifter dataset does not show a direct connection between the inner Labrador shelf and the central Labrador Sea. This is consistent with several modelling studies that investigated such exchanges between the shelf and the interior: using passive tracers in a ° model, Myers (2005) found that Baffin Bay waters remained over the Labrador shelf and slope, with very little exchange upstream of the Newfoundland shelf into the interior. Similar results were later obtained using virtual particles and passive tracers (Gillard et al., 2016; Dukhovskoy et al., 2021) or computing cross-shelf exchanges (Pennelly et al., 2019) in 0.25 to 0.08° models. Backtracking virtual particles seeded in the interior Labrador Sea over a period of 20 years, Schulze Chretien and Frajka-Williams (2018) found only 1 % of these particles originating from Baffin Bay. A few observational studies also suggested similar conclusions: using historical hydrographic data, Fratantoni and McCartney (2010) identified the first offshore pathway for Labrador shelf water just upstream of Flemish Pass. While the drifter dataset is concentrated in time, is highly biased towards the summer season, and cannot capture all possible pathways for fresh surface waters originating from Davis Strait and Hudson Strait, it offers an observational counterpart to modelling studies and mostly supports their results. The drifter data could potentially also be a useful reference for further, higher-resolution modelling studies attempting to investigate and quantify freshwater exchanges between the Labrador shelf and the interior Labrador Sea.
The present results do not however exclude the occurrence of limited exchanges between the shelf and the interior, such as suggested in Howatt et al. (2018) and Clément et al. (2023), which could be missed by the drifter dataset. Out of the 24 drifters found inshore at the Northern Labrador shelf section, one briefly entered the interior Labrador Sea before flowing back into the Labrador Current downstream. This trajectory might hint at local, time-limited exchanges between the shelf and the interior, which are not further explored here. Further studies investigating such exchanges could in particular focus on the role of winds in constraining or enhancing them: while winds over the Labrador shelf are predominantly downwelling-favourable (Yang and Chen, 2021) and contribute to constraining surface waters towards the coast, Howatt et al. (2018) identified freshwater export off the Labrador shelf south of Hamilton Bank during upwelling-favourable winds. The potential to observe such exchanges might also be affected by the drogue depth of the drifters, which are all anchored at 15 m in our dataset: using drifters anchored at 15 m and 40 cm depths, Duyck and De Jong (2021) showed that the shallower drifters were more easily driven off-shelf at Cape Farewell during export-favourable wind events.
By contrast, we observe recurrent exchanges between the coastal and shelf-break branches of the Labrador Current. Deep troughs that cut across the shelf steer waters from the shelf-break current towards the coast that then mix with the coastal current or are steered back towards the shelf break. South of 58° N, drifters that originate from the Hudson and Davis straits are not distinguishable as they flow downstream over the Labrador shelf. This is in contrast to the results from Florindo-López et al. (2020), who argue that the coastal Labrador Current stems from the Hudson Strait outflow and that it remains distinguishable from Baffin Bay waters until Seal Island at 54° N. The circulation inferred from the drifter dataset is similar to early descriptions of the Labrador Current, which highlighted the influence of troughs and saddles on the circulation (Peterson, 1987) and identified them as a potential source for the coastal branch of the Labrador Current (Colbourne et al., 1997). The drifter data do not show a direct connection between the Hudson Strait outflow and the coastal branch of the Labrador Current, but more observations would be necessary to understand this connection and the origin of the coastal branch.
Convection in the boundary current can also lead to the formation of dense waters, albeit lighter than the ones formed in the interior Labrador Sea (Pickart et al., 1997; Palter et al., 2008). Because they can be directly exported southwards, these intermediate dense waters were suggested to have a more direct impact on the overturning than waters formed in the interior, which first need to enter the boundary current via eddy exchange (Georgiou et al., 2019, 2020). Therefore, exchanges between the fresh shelf and the slope branch of the Labrador Current, where the transformation of waters to denser classes is expected to happen, could impact the formation of intermediate deep waters there and the overturning. Though the limit between the slope and shelf-break branches of the Labrador Current is difficult to identify from our dataset, Fig. 8 shows clearly different behaviours between drifters that flow over the shallower and over the deeper isobaths of the Labrador shelf and slope, suggesting limited interactions between waters found over the shelf and at the slope. This suggests that fresh waters flowing over the Labrador shelf would not immediately affect the slope branch of the Labrador Current until they reach the Newfoundland region.
4.2 Possible impact of increasing freshwater input to the Labrador shelf on the subpolar North Atlantic
Our results suggest that the influence of increasing fluxes of freshwater from the Arctic, Hudson Strait, and most of the west Greenland ice sheet would be mostly limited to the inner Labrador shelf, at least until the water reaches the Grand Banks of Newfoundland and thus is out of the Labrador Sea. This is consistent with tracer studies focusing on the fate of freshwater released from the Beaufort Gyre (Zhang et al., 2021) and the Greenland Ice Sheet (Dukhovskoy et al., 2019; Gillard et al., 2016). As they leave the shelf between Flemish Pass and the Tail of the Grand Banks, the fresh surface waters transported by the coastal and shelf-break branches of the Labrador Current mix with the salty and warm North Atlantic Current waters before continuing along their path into the subpolar North Atlantic. Increased freshwater fluxes from Davis Strait, Hudson Strait, and West Greenland glaciers could therefore lead to the formation of a salinity anomaly stemming from offshore of the Grand Banks rather than directly affect the interior Labrador Sea.
A salinity anomaly started developing offshore of the Grand Banks in 2012 (Holliday et al., 2020). The freshening was attributed to an intensified flow of Labrador Current waters off the shelf in that region following changes in regional wind patterns (Holliday et al., 2020; Fox et al., 2022; Jutras et al., 2023). Found in 2012–2016 in the Iceland Basin (Furey et al., 2023), the salinity anomaly was then advected into the Irminger Current and the interior Irminger Sea. Biló et al. (2022) argue that it led to a doubling of the stratification of the upper 1000 m in the Irminger Sea between 2017 and 2020 and was partly responsible for the suppression of deep convection in the winters of 2018 and 2019. The trajectory and impact of that salinity anomaly in the subpolar North Atlantic suggests that a freshening of the Labrador shelf due to increased freshwater input from Greenland and the Arctic could also have an indirect influence on the water properties of the upper layers and on deep convection in the Irminger and Labrador seas. The relation between increased freshwater input onto the Labrador shelf and increased freshwater fluxes off the Grand Banks would depend on several factors and likely not be linear, making the magnitude of such an anomaly difficult to estimate. For instance, a fresher shelf would increase the density gradient between the shelf and the interior, leading to an acceleration of the Labrador Current, which could also contribute to enhancing detachment at sharp bathymetric bends (Chapman, 2003; Jutras et al., 2023).
How increased freshwater input to the subpolar North Atlantic may affect the region is dependent on the origin of that freshwater: freshwater that first circulates over the East Greenland shelf, originating from Fram Strait or from Greenland, can most easily enter the Labrador Sea via the West Greenland shelf and therefore potentially have a direct impact on the salinity and stratification of its upper layer (Castelao et al., 2019). This is exemplified by the 1970s' great salinity anomaly which was attributed to increased Fram Strait outflow (Dickson et al., 1988; Häkkinen, 1993) and was suggested, together with reduced surface heat fluxes, to have contributed to a temporary shutdown of deep convection in the Labrador Sea (Lazier, 1980; Gelderloos et al., 2012). On the other hand, freshwater that stems from Baffin Bay and Davis Strait or from most of the West Greenland ice sheet mostly only enters the interior subpolar North Atlantic further downstream at the Grand Banks of Newfoundland. It then also has the potential to affect deep convection regions but indirectly as a vertically mixed salinity anomaly that circulates around the subpolar North Atlantic on a timescale of several years. Moreover, different processes that can lead to increased freshwater input to the subpolar North Atlantic from different regions operate at different timescales: while a release of the Beaufort Gyre could lead to several thousands of cubic kilometres of waters entering the region within a few years (Zhang et al., 2021; Timmermans and Toole, 2023), the accelerating melt of the Greenland Ice Sheet is a more continuous but also slower process. Additionally, icebergs and sea ice provide yet another possible pathway for Greenland and Arctic freshwater into the interior subpolar North Atlantic, as they may be more easily driven off-shelf than liquid freshwater (Marson et al., 2021; Duyck et al., 2022). Further studies could focus on understanding how all these possible freshwater pathways may differently affect the future of deep convection in the subpolar North Atlantic.
This study presents an investigation of the circulation of fresh waters originating from Baffin Bay and Hudson Bay over the Labrador shelf and into the subpolar North Atlantic, based on a surface drifter dataset spanning 1990 to 2023. It is motivated by the need to understand how increasing freshwater input from Greenland and the Arctic may affect the subpolar North Atlantic in the coming decades, as well as its impact on key processes such as deep convection in the Labrador Sea. The drifter dataset comes with several caveats: it is concentrated in time, limiting investigation of interannual variability, and it is biased towards the summer and autumn seasons. Despite these limitations, the results presented here help bridge an important gap in observational studies focused on freshwater pathways in the Labrador shelf region.
We show that waters originating from Baffin Bay and Hudson Bay mostly remain on the Labrador shelf before they reach the Newfoundland shelf and do not directly enter the interior Labrador Sea. They are exported to the subpolar North Atlantic downstream of Flemish Pass, south of the deep convection region of the Labrador Sea. Limited exchanges also take place between the shelf and slope region of the Labrador Current, suggesting only a weak direct influence of these fresh waters on convection in the boundary current.
Using the drifters, we define typical pathways between Baffin Bay and Hudson Bay and the interior subpolar North Atlantic: Baffin Bay waters first flow over the Baffin Island shelf via the Baffin Island Current. They then follow the topography to the mouth of Hudson Strait and are joined by waters from Hudson Strait and the recirculating West Greenland Current. On the Labrador shelf, most of these waters are steered towards the coast by deep canyons. A part remains in the coastal current downstream, while the rest re-enters the shelf-break Labrador Current. The major part of Baffin Bay and Hudson Bay origin waters found over the Labrador shelf then flows through Flemish Pass, and most are retroflected between the southern end of Flemish Pass and the Tail of the Grand Banks. The waters exported at the Grand Banks mix into the North Atlantic Current and spread towards the subpolar North Atlantic along its path.
Our results suggest that an increase in freshwater input to the Labrador shelf would primarily affect the volume of freshwater exported at the Grand Banks of Newfoundland rather than directly affect the interior Labrador Sea. This could possibly lead to a freshwater anomaly originating at the Grand Banks and circulating around the subpolar North Atlantic. This freshwater may then indirectly affect deep convection regions and consequently ventilation in the subpolar North Atlantic as a weakened and vertically mixed anomaly. These results highlight the importance of considering the different possible origins of freshwater in the subpolar North Atlantic, as relatively fresh waters originating from Fram Strait, Davis Strait, and Greenland follow different pathways and are influenced by different factors, which impact where, how, and how quickly they may enter the interior.
All data used in this study are publicly available.
The EGC-DrIFT dataset is available from the NIOZ dataverse repository (https://doi.org/10.25850/nioz/7b.b.ff, Duyck and De Jong, 2023b).
The sea ice dataset is the EUMETSAT OSISAF (Ocean and Sea Ice Satellite Application Facility) Global Sea Ice Concentration Data Record (https://doi.org/10.15770/EUM_SAF_OSI_0014, EUMETSAT, 2022) dataset, available from the Copernicus Climate Data Store at https://doi.org/10.24381/cds.3cd8b812 (Copernicus Climate Change Service, 2020).
The GDP dataset is available from NOAA at https://doi.org/10.25921/7ntx-z961 (Lumpkin and Centurioni, 2019). We used the ASCII files, updated through May 2023.
The bathymetry is the ETOPO2022 60 arc-second ice surface elevation, available from NOAA at https://doi.org/10.25921/fd45-gt74 (NOAA, 2022).
The scripts written to analyse the data and produce the figures are available at https://doi.org/10.5281/zenodo.14103680 (Duyck, 2024).
To perform the analysis and produce the figures, we made use of the toolboxes M_Map (https://www.eoas.ubc.ca/~rich/map.html, Pawlowicz, 2020) and jLab (https://doi.org/10.5281/zenodo.4547006, Lilly, 2024).
The supplement related to this article is available online at: https://doi.org/10.5194/os-21-241-2025-supplement.
ED and EFW conceptualized the analysis, and ED designed the methodology and performed the analysis. ED wrote the manuscript, with advice and critical feedback from EFW and NPF. All authors discussed the results and finalized the paper.
The contact author has declared that none of the authors has any competing interests.
The views and opinions expressed are those of the author(s) only and do not necessarily reflect those of the European Union or National Science Foundation. Neither the European Union nor the National Science Foundation can be held responsible for them.
Publisher's note: Copernicus Publications remains neutral with regard to jurisdictional claims made in the text, published maps, institutional affiliations, or any other geographical representation in this paper. While Copernicus Publications makes every effort to include appropriate place names, the final responsibility lies with the authors.
We thank the captains, crews, and science teams of all the research cruises that deployed the surface drifters we used in this analysis.
This research has been supported by the European Research Council (ERC) under the European Union's Horizon 2020 research and innovation programme (grant agreement no. 803140), as well as grant 2123128 from the National Science Foundation.
This paper was edited by Ilker Fer and reviewed by two anonymous referees.
Bamber, J. L., Tedstone, A. J., King, M. D., Howat, I. M., Enderlin, E. M., van den Broeke, M. R., and Noel, B.: Land Ice Freshwater Budget of the Arctic and North Atlantic Oceans: 1. Data, Methods, and Results, J. Geophys. Res.-Oceans, 123, 1827–1837, https://doi.org/10.1002/2017JC013605, 2018.
Benetti, M., Reverdin, G., Lique, C., Yashayaev, I., Holliday, N. P., Tynan, E., Torres-Valdes, S., Lherminier, P., Tréguer, P., and Sarthou, G.: Composition of freshwater in the spring of 2014 on the southern Labrador Shelf and slope, J. Geophys. Res.-Oceans, 122, 1102–1121, https://doi.org/10.1002/2016JC012244, 2017.
Biló, T. C., Straneo, F., Holte, J., and Le Bras, I. A.-A.: Arrival of New Great Salinity Anomaly Weakens Convection in the Irminger Sea, Geophys. Res. Lett., 49, e2022GL098857, https://doi.org/10.1029/2022GL098857, 2022.
Böning, C. W., Behrens, E., Biastoch, A., Getzlaff, K., and Bamber, J. L.: Emerging impact of Greenland meltwater on deepwater formation in the North Atlantic Ocean, Nat. Geosci., 9, 523–527, https://doi.org/10.1038/ngeo2740, 2016.
Buckley, M. W. and Marshall, J.: Observations, inferences, and mechanisms of the Atlantic Meridional Overturning Circulation: A review, Rev. Geophys., 54, 5–63, https://doi.org/10.1002/2015RG000493, 2016.
Buckley, M. W., Lozier, M. S., Desbruyères, D., and Evans, D. G.: Buoyancy forcing and the subpolar Atlantic meridional overturning circulation, Philos. T. R. Soc. A, 381, 20220181, https://doi.org/10.1098/rsta.2022.0181, 2023.
Castelao, R. M., Luo, H., Oliver, H., Rennermalm, A. K., Tedesco, M., Bracco, A., Yager, P. L., Mote, T. L., and Medeiros, P. M.: Controls on the Transport of Meltwater From the Southern Greenland Ice Sheet in the Labrador Sea, J. Geophys. Res.-Oceans, 124, 3551–3560, https://doi.org/10.1029/2019JC015159, 2019.
Centurioni, L. R., Turton, J., Lumpkin, R., Braasch, L., Brassington, G., Chao, Y., Charpentier, E., Chen, Z., Corlett, G., Dohan, K., Donlon, C., Gallage, C., Hormann, V., Ignatov, A., Ingleby, B., Jensen, R., Kelly-Gerreyn, B. A., Koszalka, I. M., Lin, X., Lindstrom, E., Maximenko, N., Merchant, C. J., Minnett, P., O'Carroll, A., Paluszkiewicz, T., Poli, P., Poulain, P.-M., Reverdin, G., Sun, X., Swail, V., Thurston, S., Wu, L., Yu, L., Wang, B., and Zhang, D.: Global in situ Observations of Essential Climate and Ocean Variables at the Air–Sea Interface, Front. Mar. Sci., 6, 419, https://doi.org/10.3389/fmars.2019.00419, 2019.
Chapman, D. C.: Separation of an Advectively Trapped Buoyancy Current at a Bathymetric Bend, J. Phys. Oceanogr., 33, 1108–1121, https://doi.org/10.1175/1520-0485(2003)033<1108:SOAATB>2.0.CO;2, 2003.
Clément, L., Frajka-Williams, E., von Oppeln-Bronikowski, N., Goszczko, I., and deYoung, B.: Cessation of Labrador Sea Convection Triggered by Distinct Fresh and Warm (Sub)Mesoscale Flows, J. Phys. Oceanogr., 53, 1959–1977, https://doi.org/10.1175/JPO-D-22-0178.1, 2023.
Colbourne, E., deYoung, B., Narayanan, S., and Helbig, J.: Comparison of hydrography and circulation on the Newfoundland Shelf during Pages 1990–1993 with the long-term mean, Can. J. Fish. Aquat. Sci., 54, 68–80, https://doi.org/10.1139/f96-156, 1997.
Copernicus Climate Change Service (C3S): Sea ice concentration daily gridded data from 1978 to present derived from satellite observations, Copernicus Climate Change Service (C3S) Climate Data Store (CDS) [data set], https://doi.org/10.24381/cds.3cd8b812, 2020.
Cuny, J., Rhines, P. B., Niiler, P. P., and Bacon, S.: Labrador Sea Boundary Currents and the Fate of the Irminger Sea Water, J. Phys. Oceanogr., 32, 627–647, https://doi.org/10.1175/1520-0485(2002)032<0627:LSBCAT>2.0.CO;2, 2002.
Cuny, J., Rhines, P. B., and Ron Kwok: Davis Strait volume, freshwater and heat fluxes, Deep-Sea Res. Pt. I, 52, 519–542, https://doi.org/10.1016/j.dsr.2004.10.006, 2005.
Curry, B., Lee, C. M., Petrie, B., Moritz, R. E., and Kwok, R.: Multiyear Volume, Liquid Freshwater, and Sea Ice Transports through Davis Strait, 2004–10, J. Phys. Oceanogr., 44, 1244–1266, https://doi.org/10.1175/JPO-D-13-0177.1, 2014.
Cyr, F. and Galbraith, P. S.: A climate index for the Newfoundland and Labrador shelf, Earth Syst. Sci. Data, 13, 1807–1828, https://doi.org/10.5194/essd-13-1807-2021, 2021.
de Jong, M. F., van Aken, H. M., Våge, K., and Pickart, R. S.: Convective mixing in the central Irminger Sea: 2002–2010, Deep-Sea Res. Pt. I, 63, 36–51, https://doi.org/10.1016/j.dsr.2012.01.003, 2012.
Dickson, R. R., Meincke, J., Malmberg, S.-A., and Lee, A. J.: The “great salinity anomaly” in the Northern North Atlantic 1968–1982, Prog. Oceanogr., 20, 103–151, https://doi.org/10.1016/0079-6611(88)90049-3, 1988.
Dukhovskoy, D. S., Yashayaev, I., Proshutinsky, A., Bamber, J. L., Bashmachnikov, I. L., Chassignet, E. P., Lee, C. M., and Tedstone, A. J.: Role of Greenland Freshwater Anomaly in the Recent Freshening of the Subpolar North Atlantic, J. Geophys. Res.-Oceans, 124, 3333–3360, https://doi.org/10.1029/2018JC014686, 2019.
Dukhovskoy, D. S., Yashayaev, I., Chassignet, E. P., Myers, P. G., Platov, G., and Proshutinsky, A.: Time Scales of the Greenland Freshwater Anomaly in the Subpolar North Atlantic, J. Climate, 34, 8971–8987, https://doi.org/10.1175/JCLI-D-20-0610.1, 2021.
Duyck, E.: Code – Circulation of Baffin Bay and Hudson Bay waters on the Labrador Shelf and into the subpolar North Atlantic, Zenodo [code], https://doi.org/10.5281/zenodo.14103680, 2024.
Duyck, E. and De Jong, M. F.: Circulation Over the South-East Greenland Shelf and Potential for Liquid Freshwater Export: A Drifter Study, Geophys. Res. Lett., 48, e2020JB020886, https://doi.org/10.1029/2020GL091948, 2021.
Duyck, E. and De Jong, M. F.: Cross-Shelf Exchanges Between the East Greenland Shelf and Interior Seas, J. Geophys. Res.-Oceans, 128, e2023JC019905, https://doi.org/10.1029/2023JC019905, 2023a.
Duyck, E. and De Jong, M. F.: Full EGC-DrIFT 6h interpolated dataset, NIOZ [data set], https://doi.org/10.25850/nioz/7b.b.ff, 2023b.
Duyck, E., Gelderloos, R., and de Jong, M. F.: Wind-Driven Freshwater Export at Cape Farewell, J. Geophys. Res.-Oceans, 127, e2021JC018309, https://doi.org/10.1029/2021JC018309, 2022.
EUMETSAT: Ocean and Sea Ice Satellite Application Facility, Global sea ice concentration interim climate data record 2021-onwards (v3.0, 2022), OSI-430-a, [data set], https://doi.org/10.15770/EUM_SAF_OSI_0014, 2022.
Florindo-López, C., Bacon, S., Aksenov, Y., Chafik, L., Colbourne, E., and Holliday, N. P.: Arctic Ocean and Hudson Bay Freshwater Exports: New Estimates from Seven Decades of Hydrographic Surveys on the Labrador Shelf, J. Climate, 33, 8849–8868, https://doi.org/10.1175/JCLI-D-19-0083.1, 2020.
Fox, A. D., Handmann, P., Schmidt, C., Fraser, N., Rühs, S., Sanchez-Franks, A., Martin, T., Oltmanns, M., Johnson, C., Rath, W., Holliday, N. P., Biastoch, A., Cunningham, S. A., and Yashayaev, I.: Exceptional freshening and cooling in the eastern subpolar North Atlantic caused by reduced Labrador Sea surface heat loss, Ocean Sci., 18, 1507–1533, https://doi.org/10.5194/os-18-1507-2022, 2022.
Fratantoni, D. M.: North Atlantic surface circulation during the 1990's observed with satellite-tracked drifters, J. Geophys. Res.-Oceans, 106, 22067–22093, https://doi.org/10.1029/2000JC000730, 2001.
Fratantoni, P. S. and McCartney, M. S.: Freshwater export from the Labrador Current to the North Atlantic Current at the Tail of the Grand Banks of Newfoundland, Deep-Sea Res. Pt. I, 57, 258–283, https://doi.org/10.1016/j.dsr.2009.11.006, 2010.
Fratantoni, P. S. and Pickart, R. S.: The Western North Atlantic Shelfbreak Current System in Summer, J. Phys. Oceanogr., 37, 2509–2533, https://doi.org/10.1175/JPO3123.1, 2007.
Furey, H. H., Foukal, N. P., Anderson, A., and Bower, A. S.: Investigation of the Source of Iceland Basin Freshening: Virtual Particle Tracking with Satellite-Derived Geostrophic Surface Velocities, Remote Sens., 15, 5711, https://doi.org/10.3390/rs15245711, 2023.
Gelderloos, R., Straneo, F., and Katsman, C. A.: Mechanisms behind the Temporary Shutdown of Deep Convection in the Labrador Sea: Lessons from the Great Salinity Anomaly Years 1968–71, J. Climate, 25, 6743–6755, https://doi.org/10.1175/JCLI-D-11-00549.1, 2012.
Georgiou, S., van der Boog, C. G., Brüggemann, N., Ypma, S. L., Pietrzak, J. D., and Katsman, C. A.: On the interplay between downwelling, deep convection and mesoscale eddies in the Labrador Sea, Ocean Model., 135, 56–70, https://doi.org/10.1016/j.ocemod.2019.02.004, 2019.
Georgiou, S., Ypma, S. L., Brüggemann, N., Sayol, J.-M., Pietrzak, J. D., and Katsman, C. A.: Pathways of the water masses exiting the Labrador Sea: The importance of boundary–interior exchanges, Ocean Model., 150, 101623, https://doi.org/10.1016/j.ocemod.2020.101623, 2020.
Gillard, L. C., Hu, X., Myers, P. G., and Bamber, J. L.: Meltwater pathways from marine terminating glaciers of the Greenland ice sheet, Geophys. Res. Lett., 43, 10873–10882, https://doi.org/10.1002/2016GL070969, 2016.
Gonçalves Neto, A., Palter, J. B., Xu, X., and Fratantoni, P.: Temporal Variability of the Labrador Current Pathways Around the Tail of the Grand Banks at Intermediate Depths in a High-Resolution Ocean Circulation Model, J. Geophys. Res.-Oceans, 128, e2022JC018756, https://doi.org/10.1029/2022JC018756, 2023.
Haine, T. W. N., Curry, B., Gerdes, R., Hansen, E., Karcher, M., Lee, C., Rudels, B., Spreen, G., de Steur, L., Stewart, K. D., and Woodgate, R.: Arctic freshwater export: Status, mechanisms, and prospects, Global Planet. Change, 125, 13–35, https://doi.org/10.1016/j.gloplacha.2014.11.013, 2015.
Häkkinen, S.: An Arctic source for the great salinity anomaly: A simulation of the Arctic ice-ocean system for 1955–1975, J. Geophys. Res.-Oceans, 98, 16397–16410, https://doi.org/10.1029/93JC01504, 1993.
Holliday, N. P., Bersch, M., Berx, B., Chafik, L., Cunningham, S., Florindo-López, C., Hátún, H., Johns, W., Josey, S. A., Larsen, K. M. H., Mulet, S., Oltmanns, M., Reverdin, G., Rossby, T., Thierry, V., Valdimarsson, H., and Yashayaev, I.: Ocean circulation causes the largest freshening event for 120 years in eastern subpolar North Atlantic, Nat. Commun., 11, 585, https://doi.org/10.1038/s41467-020-14474-y, 2020.
Howatt, T., Palter, J. B., Matthews, J. B. R., deYoung, B., Bachmayer, R., and Claus, B.: Ekman and Eddy Exchange of Freshwater and Oxygen across the Labrador Shelf Break, J. Phys. Oceanogr., 48, 1015–1031, https://doi.org/10.1175/JPO-D-17-0148.1, 2018.
Jutras, M., Dufour, C. O., Mucci, A., and Talbot, L. C.: Large-scale control of the retroflection of the Labrador Current, Nat. Commun., 14, 2623, https://doi.org/10.1038/s41467-023-38321-y, 2023.
Lazier, J. R. N.: Oceanographic conditions at Ocean Weather Ship Bravo, 1964–1974, Atmos. Ocean, 18, 227–238, https://doi.org/10.1080/07055900.1980.9649089, 1980.
Lazier, J. R. N. and Wright, D. G.: Annual Velocity Variations in the Labrador Current, J. Phys. Oceanogr., 23, 659–678, https://doi.org/10.1175/1520-0485(1993)023<0659:AVVITL>2.0.CO;2, 1993.
LeBlond, P. H., Osborn, T. R., Hodgins, D. O., Goodman, R., and Metge, M.: Surface circulation in the Western Labrador Sea, Deep-Sea Res. Pt. A, 28, 683–693, https://doi.org/10.1016/0198-0149(81)90129-1, 1981.
Lilly, J. M.: jLab: A data analysis package for Matlab, v.1.7.3, Zenodo [code], https://doi.org/10.5281/zenodo.4547006, 2024.
Lilly, J. M., Rhines, P. B., Schott, F., Lavender, K., Lazier, J., Send, U., and D'Asaro, E.: Observations of the Labrador Sea eddy field, Prog. Oceanogr., 59, 75–176, https://doi.org/10.1016/j.pocean.2003.08.013, 2003.
Lin, P., Pickart, R. S., Heorton, H., Tsamados, M., Itoh, M., and Kikuchi, T.: Recent state transition of the Arctic Ocean's Beaufort Gyre, Nat. Geosci., 16, 485–491, https://doi.org/10.1038/s41561-023-01184-5, 2023.
Loder, J., Petrie, B., and Gawarkiewicz, G.: The Coastal Ocean off Northeastern North America: A Large-Scale View, The Sea, edited by: Robinson, A. R. and Brink, K. H., Regional Studies and Syntheses, Vol. 11, 105–133, Harvard University Press, ISBN 9780674017412, 1998.
Lozier, M. S.: Overturning in the subpolar North Atlantic: a review, Philos. T. R. Soc. A, 381, 20220191, https://doi.org/10.1098/rsta.2022.0191, 2023.
Lumpkin, R. and Centurioni, L.: Global Drifter Program quality-controlled 6-hour interpolated data from ocean surface drifting buoys, NOAA National Centers for Environmental Information [data set], https://doi.org/10.25921/7ntx-z961, 2019.
Luo, H., Castelao, R. M., Rennermalm, A. K., Tedesco, M., Bracco, A., Yager, P. L., and Mote, T. L.: Oceanic transport of surface meltwater from the southern Greenland ice sheet, Nat. Geosci., 9, 528–532, https://doi.org/10.1038/ngeo2708, 2016.
Marsh, R., Desbruyères, D., Bamber, J. L., de Cuevas, B. A., Coward, A. C., and Aksenov, Y.: Short-term impacts of enhanced Greenland freshwater fluxes in an eddy-permitting ocean model, Ocean Sci., 6, 749–760, https://doi.org/10.5194/os-6-749-2010, 2010.
Marson, J. M., Gillard, L. C., and Myers, P. G.: Distinct ocean responses to Greenland's liquid runoff and iceberg melt, J. Geophys. Res.-Oceans, 126, e2021JC017542, https://doi.org/10.1029/2021JC017542, 2021.
Myers, P. G.: Impact of freshwater from the Canadian Arctic Archipelago on Labrador Sea Water formation, Geophys. Res. Lett., 32, L06605, https://doi.org/10.1029/2004GL022082, 2005.
NOAA: National Centers for Environmental Information: ETOPO 2022 15 Arc-Second Global Relief Model, NOAA National Centers for Environmental Information [data set], https://doi.org/10.25921/fd45-gt74, 2022.
Palter, J. B., Lozier, M. S., and Lavender, K. L.: How Does Labrador Sea Water Enter the Deep Western Boundary Current?, J. Phys. Oceanogr., 38, 968–983, https://doi.org/10.1175/2007JPO3807.1, 2008.
Pawlowicz, R.: M_Map: A mapping package for MATLAB, v1.4m, MATLAB [code], https://www.eoas.ubc.ca/~rich/map.html (last access: 24 January 2025), 2020.
Pennelly, C., Hu, X., and Myers, P. G.: Cross-Isobath Freshwater Exchange Within the North Atlantic Subpolar Gyre, J. Geophys. Res.-Oceans, 124, 6831–6853, https://doi.org/10.1029/2019JC015144, 2019.
Peterson, I.: A snapshot of the Labrador current inferred from ice-floe movement in NOAA satellite imagery, Atmos. Ocean, 25, 402–415, https://doi.org/10.1080/07055900.1987.9649283, 1987.
Petrie, B. and Anderson, C.: Circulation on the newfoundland continental shelf, Atmos. Ocean, 21, 207–226, https://doi.org/10.1080/07055900.1983.9649165, 1983.
Petrie, B. and Drinkwater, K.: Temperature and salinity variability on the Scotian Shelf and in the Gulf of Maine 1945–1990, J. Geophys. Res.-Oceans, 98, 20079–20089, https://doi.org/10.1029/93JC02191, 1993.
Pickart, R. S., Spall, M. A., and Lazier, J. R. N.: Mid-depth ventilation in the western boundary current system of the sub-polar gyre, Deep-Sea Res. Pt. I, 44, 1025–1054, https://doi.org/10.1016/S0967-0637(96)00122-7, 1997.
Reverdin, G., Niiler, P. P., and Valdimarsson, H.: North Atlantic Ocean surface currents, J. Geophys. Res.-Oceans, 108, 2-1–2-21, https://doi.org/10.1029/2001JC001020, 2003.
Rhein, M., Steinfeldt, R., Kieke, D., Stendardo, I., and Yashayaev, I.: Ventilation variability of Labrador Sea Water and its impact on oxygen and anthropogenic carbon: a review, Philos. T. R. Soc. A, 375, 20160321, https://doi.org/10.1098/rsta.2016.0321, 2017.
Ridenour, N. A., Straneo, F., Holte, J., Gratton, Y., Myers, P. G., and Barber, D. G.: Hudson Strait Inflow: Structure and Variability, J. Geophys. Res.-Oceans, 126, e2020JC017089, https://doi.org/10.1029/2020JC017089, 2021.
Schiller-Weiss, I., Martin, T., and Schwarzkopf, F. U.: Emerging Influence of Enhanced Greenland Melting on Boundary Currents and Deep Convection Regimes in the Labrador and Irminger Seas, Geophys. Res. Lett., 51, e2024GL109022, https://doi.org/10.1029/2024GL109022, 2024.
Schulze Chretien, L. M. and Frajka-Williams, E.: Wind-driven transport of fresh shelf water into the upper 30 m of the Labrador Sea, Ocean Sci., 14, 1247–1264, https://doi.org/10.5194/os-14-1247-2018, 2018.
Shaw, J.-L. and Galbraith, P. S.: Climatology of Transport in the Strait of Belle Isle, J. Geophys. Res.-Oceans, 128, e2022JC019084, https://doi.org/10.1029/2022JC019084, 2023.
Shu, Q., Qiao, F., Song, Z., Zhao, J., and Li, X.: Projected Freshening of the Arctic Ocean in the 21st Century, J. Geophys. Res.-Oceans, 123, 9232–9244, https://doi.org/10.1029/2018JC014036, 2018.
Smith, E. H., Soule, F. M., and Mosby, O.: The Marion and General Greene Expeditions to the Davis Strait and Labrador Sea Under the Direction of the United States Coast Guard, 1928–1931–1933–1934–1935 – Scientific Results Part 2: Physical Oceanography, U.S. Govt. Print. Off., https://doi.org/10.5962/bhl.title.10182, 1937.
Solodoch, A., McWilliams, J. C., Stewart, A. L., Gula, J., and Renault, L.: Why Does the Deep Western Boundary Current “Leak” around Flemish Cap?, J. Phys. Oceanogr., 50, 1989–2016, https://doi.org/10.1175/JPO-D-19-0247.1, 2020.
Steinfeldt, R., Rhein, M., Bullister, J. L., and Tanhua, T.: Inventory changes in anthropogenic carbon from 1997–2003 in the Atlantic Ocean between 20° S and 65° N, Global Biogeochem. Cy., 23, https://doi.org/10.1029/2008GB003311, 2009.
Straneo, F. and Saucier, F.: The outflow from Hudson Strait and its contribution to the Labrador Current, Deep-Sea Res. Pt. I, 55, 926–946, https://doi.org/10.1016/j.dsr.2008.03.012, 2008.
Swift, J. H. and Aagaard, K.: Seasonal transitions and water mass formation in the Iceland and Greenland seas, Deep-Sea Res. Pt. A, 28, 1107–1129, https://doi.org/10.1016/0198-0149(81)90050-9, 1981.
The IMBIE team: Mass balance of the Greenland Ice Sheet from 1992 to 2018, Nature, 579, 233–239, https://doi.org/10.1038/s41586-019-1855-2, 2020.
Timmermans, M.-L. and Toole, J. M.: The Arctic Ocean's Beaufort Gyre, Annu. Rev. Mar. Sci., 15, 223–248, https://doi.org/10.1146/annurev-marine-032122-012034, 2023.
Yang, J. and Chen, K.: The role of wind stress in driving the along-shelf flow in the northwest Atlantic Ocean, J. Geophys. Res.-Oceans, 126, e2020JC016757, https://doi.org/10.1029/2020JC016757, 2021.
Yang, Q., Dixon, T. H., Myers, P. G., Bonin, J., Chambers, D., van den Broeke, M. R., Ribergaard, M. H., and Mortensen, J.: Recent increases in Arctic freshwater flux affects Labrador Sea convection and Atlantic overturning circulation, Nat. Commun., 7, 10525, https://doi.org/10.1038/ncomms10525, 2016.
Zhang, J., Weijer, W., Steele, M., Cheng, W., Verma, T., and Veneziani, M.: Labrador Sea freshening linked to Beaufort Gyre freshwater release, Nat. Commun., 12, 1229, https://doi.org/10.1038/s41467-021-21470-3, 2021.